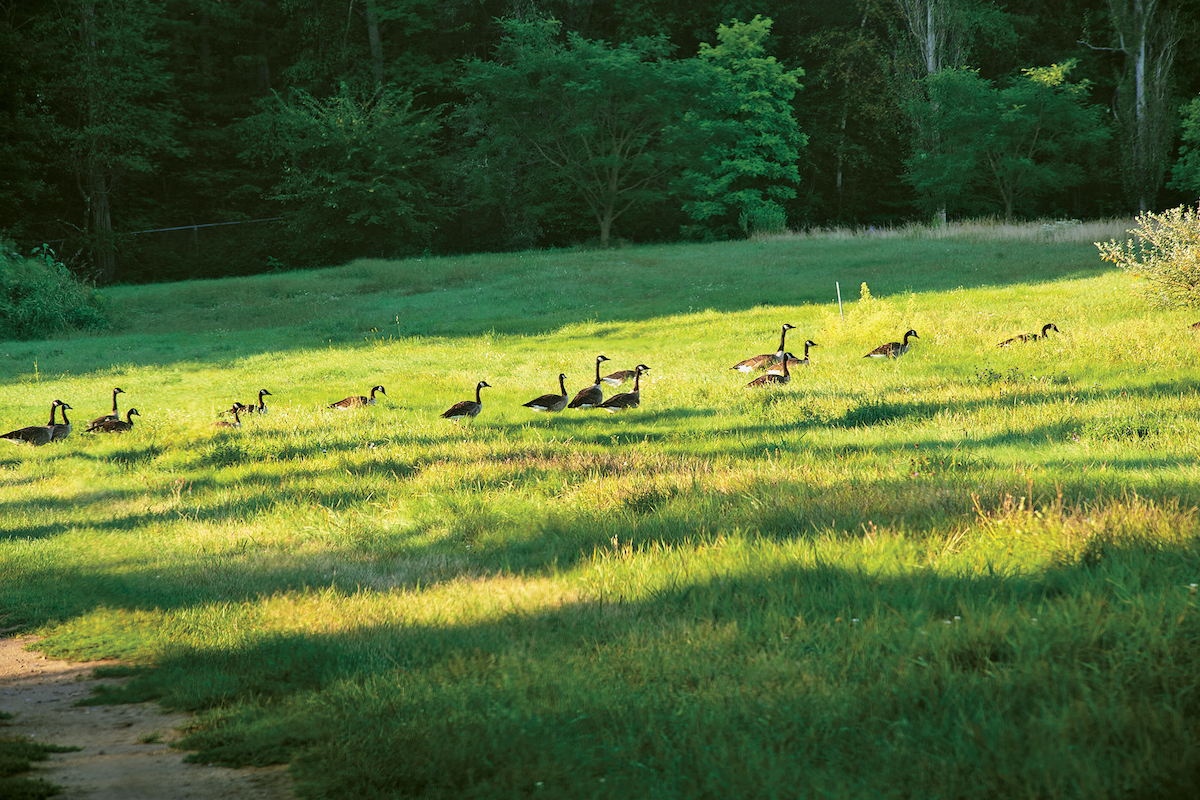
Fish and Wildlife in Vermont
Download chapter PDF and figures
Authors
George Ni
Biology Department, University of Vermont, Burlington, VT 05405
Joshua Morse
Rubenstein School of Natural Resources and Environment and Gund Institute, University of Vermont, Burlington, VT 05405
Steve Faccio
Senior Conservation Biologist, Vermont Center for Ecostudies, White River Junction, VT 05001
Citation
Ni, G., Morse, J., Faccio, S. 2021. Fish and Wildlife in Vermont. In Galford, G.L., Faulkner, J. et al. (Eds), The Vermont Climate Assessment 2021. Burlington, Vermont: Gund Institute for Environment at the University of Vermont. DOI: 10.18125/kowgvg.
Table of Contents
- 4.1 Key Messages
- 4.2 Background
- 4.3 Insects: Pollinators and Forest Pests
- 4.4 Birds
- 4.5 Bats
- 4.6 Large Ungulates (Moose and Deer)
- 4.7 Aquatic Species
- 4.8 Traceable Accounts
- 4.9 References
4.1 Key Messages
- As climate change worsens, 92 bird species of Vermont, including the iconic common loon and hermit thrush, are expected to disappear from the landscape within the next 25 years.
- Increasing warming trends are expected to result in an increase in white-tailed deer population and a mirrored decrease in moose population, which may have long-term impacts on Vermont’s forest composition. Managing social systems (e.g. hunting) to account for changing public tolerance and demand for deer may provide one avenue to minimize this risk if undertaken proactively.
- As warming trends reduce the severity of winters, the subsequent warming waters will have adverse effects on lake and river systems, including increased risk for harmful algal blooms (HABs) and reduced overall biodiversity and health in lake ecosystems.
4.2 Background
Anthropogenic climate change has had a warming influence on the planet since the 1750s that has resulted in increased global mean temperature. As a result, Earth has seen shifts in annual precipitation, average seasonality, and a host of other changes. These shifts affect the environment in several ways, and the complex interactions that occur can often seem opaque. This is especially true when considering how climate change may affect organisms other than humans. Without proper monitoring and study of organisms, it is difficult to parse out the full effect that climate change may have on different species, as well as the full impact that policy plans or management strategies may have as a proactive plan to buffer those shifts. Current research is attempting to address these issues, and many important interactions have been documented and observed to link climate change to organism behavior and survival. A few of these interactions are listed below as they are often important indicators for fish and wildlife.
4.2.1 Annual Precipitation and Rainfall Events
Reports and monitoring efforts across Vermont have recorded ever-increasing levels of precipitation annually since the early 1900s, with average annual precipitation across all seasons seeing significant positive trends. Since 1960, summer precipitation in Vermont has seen the fastest increase with 0.5 inches per decade, winter and fall each with 0.3 inches per decade, and spring with 0.2 inches per decade (see Climate Change in Vermont chapter). This impacts a variety of aquatic and terrestrial organisms that rely on Vermont’s riverine and lake systems for shelter, reproductive cycles, and resources.
4.2.2 Increasing Annual Mean Temperature and Winter/Spring Seasonality
Based on current climate projections, winter temperatures will gradually increase, reducing winter severity and shortening the winter season itself. This will have a negative effect on the total ice cover of water bodies. Changes in winter seasonality have also resulted in shifts in animal community structures to more warm-adapted species. Vermont’s annual average temperature has increased by almost 2℉ since 1900, but winter temperatures have increased 2.5 times faster than annual temperatures over the last 60 years (VCA Physical Climate Chapter).
4.2.3 Land-Use Changes
As organisms are intrinsically tied to their habitat, anthropogenic climate change through urbanization, forest clearings, and reforestation post-agricultural abandonment has a direct effect on their overall fitness and ability to survive in their natural habitats. Increasing urbanization in montane areas and undeveloped land leads to issues of habitat loss and habitat fragmentation, both of which heavily impact biodiversity and ecological processes within a given ecosystem. This applies to both terrestrial and aquatic species, with road and dam infrastructures resulting in a variety of consequences for vulnerable species.
4.2.4 Phenological Interactions
In response to large-scale warming of their environments, many organisms are forced to shift their distributions and seasonal interactions, such as phenological events of flowering, breeding, migrating, or growing. Vermont is already experiencing increased temperatures, with average winter temperatures increasing 3.08℉ since the 1960s (see Climate Change in Vermont chapter). Historical observations show the freeze-free period (number of consecutive days with minimum temperature above 28ºF) has increased by 9.0 days per decade since the 1990s (see Climate Change in Vermont chapter). Increases in winter temperature and overall reduction in winter severity can lead to dramatic shifts in seasonality, which can be a considerable obstacle for wildlife with life histories that closely follow local climate patterns. Issues with phenological interactions may occur in species with narrow breeding times or migration patterns, as they are often reliant on complex interactions with organisms from other trophic levels in order to function properly. Migratory birds rely on booms in insect populations during the spring to recover from the physical strains of traveling and to support reproductive strategies. When the timing of insect emergence does not coincide with the arrival of migratory birds, this can often lead to phenological mismatch, where those birds are unable to take full advantage of the insect boom and may starve or fail to reproduce. As such, birds, insects, and fish are often susceptible to shifts in phenology.
4.3 Insects: Pollinators and Forest Pests
Insects are often overlooked when studying climate change, whether that is due to the difficulties in gathering appropriate data on such small organisms, or preconceptions that insects play less of a role or are less valuable compared to larger, more charismatic species like birds and larger ungulates (Polgar et al., 2013). In fact, insects can provide a variety of ecosystem functions that largely go unnoticed by humans. Gardens and fields are heavily reliant on pollinators to initiate the growth of flowers and fruit-bearing plants, and caterpillars are the primary source of food for migratory birds recovering after their long flights. Insects are also extremely responsive to changes in their environment, and climate change effects have drastic impacts on their survival. In order to fully capture the extent of climate change, it is important to acknowledge the widespread effects that it will have and how it might affect humans.
4.3.1 Pollinators: Bees
Worldwide, bees are considered one of the most important pollinators, as they provide crucial pollination to fruit-bearing plants and flowers, and have an immense impact on agriculture and food systems. Within Vermont, close to 275 species of bees have been found, with most of them “solitary bees” that do not have colonies like honey bees but still serve as pollinators. These native bees are often more valuable as pollinators compared to the more well-known honey bee due to their wider visitation range and pollination activity. Their contribution has widespread effects on wildlife that rely on fruits, such as turkey, moose, and deer.
Concerns for bees stem from recent reports of commercial honey bee colony collapses. Current studies in Vermont have corroborated many of the indications by population trends: that pollinators are declining nationwide and are at risk of disappearing. A bumble bee survey conducted in 2012 found that of the 17 major bumble bee species commonly found in Vermont, 4 were not detected and 4 showed significant declines (Nicholson et al., 2017). One major factor for these declines is anthropogenic impacts on bee species, especially agriculture.
Agricultural practices can disrupt bee populations on multiple different scales, from habitat degradation, farm management practices, pathogens, and climate change. Bee communities are heavily influenced by the surrounding landscape and the management practices employed there. Intensive farm management practices can often intensify the negative effects of landscape simplification (Nicholson et al., 2017). Less intensively managed farms had more instances of bee visitations and more diverse communities of bee species.
On a larger scale, climate change is also having adverse effects on bees. Warming temperature trends are predicted to affect bees through phenological mismatch. As the warming seasons result in earlier spring times, there may be a disconnect that occurs between the bees and the plants, as the bees may become active later in the season compared to the plants they would have visited (Memmott et al., 2007). Figure 4-1 highlights the impact that warmer temperatures under climate change may have on phenological mismatching.
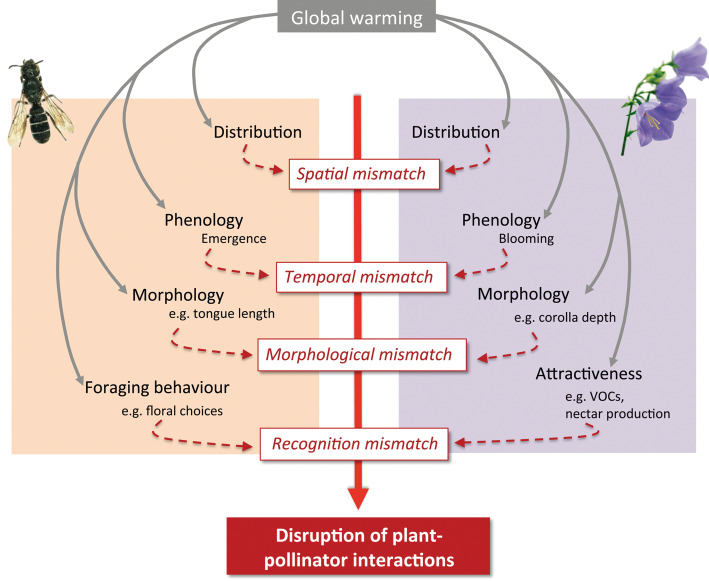
4.3.2 Forest Pests
While insects often provide beneficial ecosystem functions, there are of course examples of insects that are seen as destructive or detrimental to an ecosystem’s health. These are important to acknowledge so that forest managers can proactively develop plans and practices to address these problems.
Hemlock woolly adelgid (HWA) and spruce budworm are seen as forest pests by wildlife and forest managers, especially given the invasive nature of the hemlock woolly adelgid. They are particularly destructive in North America as they have no natural predators or checks to keep them from running rampant.
HWA is a forest pest that feeds solely on the sap of hemlock and spruce trees. Without the presence of predators, they are able to quickly expand and severely hamper the hemlock tree population. Not only that, but the HWA is also able to actively feed and develop during winter, which is not common for insects. This acts as a double-edged sword, as they are extremely susceptible to extreme weather events and winter temperatures. As such, overwintering mortality due to minimum winter temperatures is the main factor in determining HWA density and spread. Observations in Vermont indicate that average winter temperatures are warming 0.5℉ per decade for the last three decades and that the number of very cold winter days (maximum temperature below 0℉) has been decreasing 3 days per decade since the 1990s (see Climate Change in Vermont chapter). These trends suggest winter survival of the HWA may increase along with increases in population growth rates towards northeastern edges of Eastern Hemlock trees (McAvoy et al., 2017).
Vermont is committed to studying the spread and distribution of invasive forest pests, with monitoring programs for a variety of the most common invasives and implementation of management strategies. Organizations such as Vermont Invasives provide valuable information to the public about aquatic and terrestrial invasive species while also giving community members the opportunity to participate through citizen science—contributing information and reporting sightings of invasives themselves.
4.4 Birds
4.4.1 Background
Birds represent a critical source of biodiversity in Vermont and provide a number of ecological functions that affect humans. Not only are birds a source of entertainment for birdwatchers, but as seed-dispersers and agents of natural pest control, they are vital to the ecosystem. When thinking about climate change impacts on birds, a critical issue is the impact it may have on phenological timing. This is especially true for migratory birds. Breeding migratory birds must have precise timing of their arrival to spring feeding grounds, as individuals arriving too early may be risking disadvantageous conditions or a constrained amount of food resources, while those arriving too late face difficulties in finding accessible mates or suitable breeding territories. To properly match their migration to environmental conditions, birds often synchronize their timing based on environmental cues like temperature and photoperiod. Figures 4-3 and 4-4 highlight this relationship, illustrating a tightly-knit association between arrival date of migrating birds and the trajectory of seasonal temperatures per year.
Researchers at the Vermont Center for Ecostudies have tracked an alarming trend of declining aerial insectivore bird species. They have noted a 2.5% annual decrease in bird counts in their study sites, equating to a 45% drop in their relative abundance over 25 years (Figure 4-2). With such a massive drop in abundance in a relatively diverse guild, it is likely that these declines represent broadscale changes—whether that be to insect phenology, pesticide use, or loss of insect habitats—and resultant declines in aerial insectivore abundance (Faccio et al. 2017).
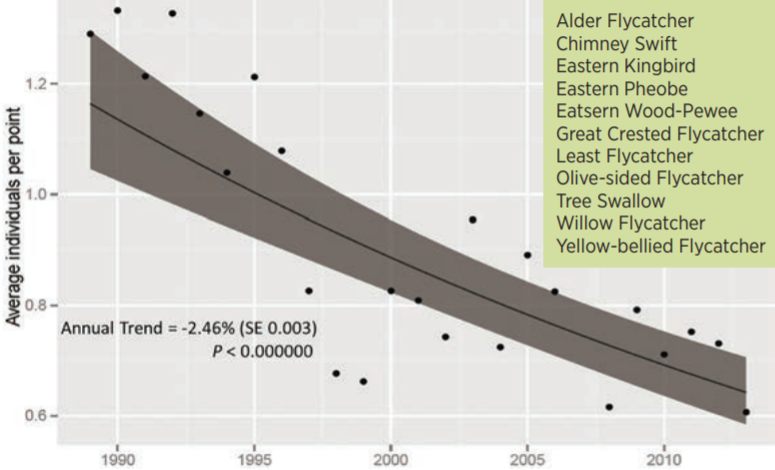
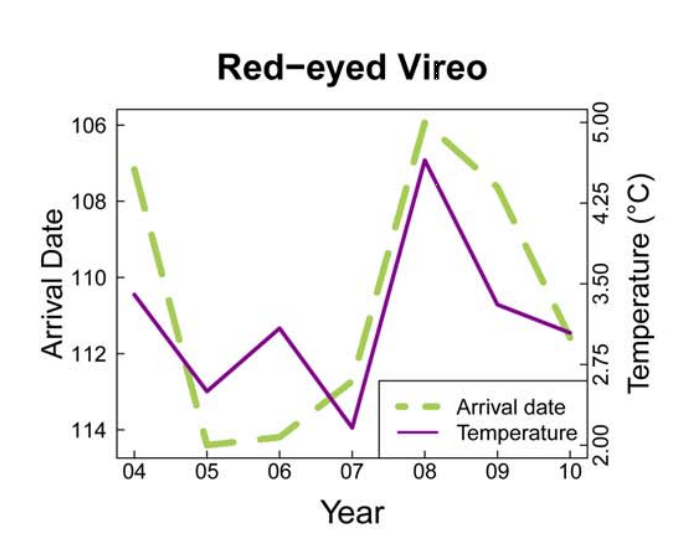
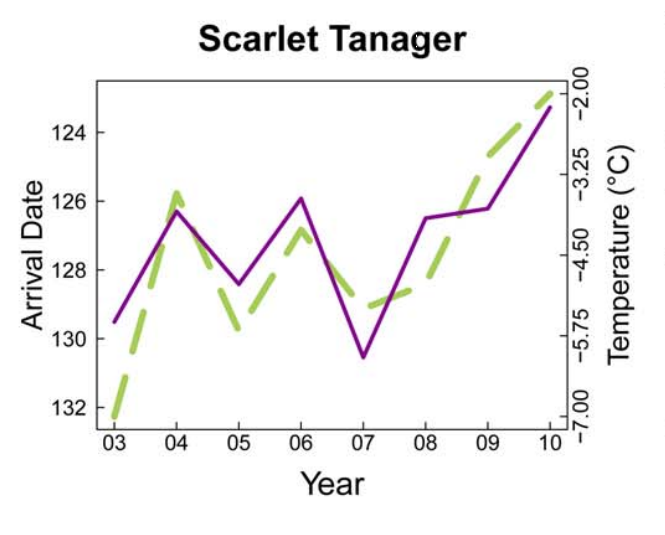
4.4.2 Seasonality
Increases in the average spring temperature in Vermont (+0.3℉ since the 1990s) can have big impacts on plants. Changes in spring seasonality can jump-start the spring growing season, with a wide variability in how species have reacted to this change in timing in Vermont (Hurlbert and Liang, 2012). This variability can lead to phenological mismatch, where the phenological timings of interacting species shift at different rates. In migrating birds, this asynchrony can result in widespread starvation and decreased fitness of species if they migrate at a time when their food sources are either not available due to growth patterns, or have already emerged and were eaten by other bird consumers that had migrated at the correct time. Figures 4-3 and 4-4 highlight this pattern, illustrating how closely the arrival dates of different bird species coincide with the temperature, and how variable the timing can be.
Although many migratory songbirds are at risk of starvation and loss of breeding habitats due to warming temperature trends, there are cases of birds that could benefit from extended breeding seasons. Birds with the ability to lay multiple broods, such as the black-throated blue warbler, can sometimes utilize the extended breeding season for population growth and recruitment (Townsend et al, 2013). Yet nothing is straightforward—the black-throated blue warbler may be hindered by loss of hobblebush habitat that is maintained by browsing moose which are declining.
Vermont’s winters are warming rapidly, with drastic changes to the structure of winter bird communities. As bird populations respond to warming climates through slow poleward migrations, overwintering bird communities are populated by increasing numbers of warm-adapted species across North America (Prince and Zuckerberg, 2015). This raises concerns of how this shift in community structure may impact the environment and landscape over time.
4.4.3 Habitats and Range Shifts
Researchers are observing a trend in poleward migrations of birds due to warming temperatures and landscape changes that could have drastic consequences for threatened species. In Vermont, the most well-known example is the Bicknell’s thrush (Catharus bicknelli), a migratory bird with heavily restricted ranges that is one of the region’s highest conservation priorities. As it only lives in mountainous habitats at high elevations with dense balsam firs, it is particularly vulnerable, and bioclimatic models predict over 50% of its suitable habitat will be lost by 2050 (Cadieux et al., 2019). Not only are warming climates pushing montane spruce forests towards extinction (see Climate Change in Forests chapter), but anthropogenic impacts such as ski areas, towers, and turbines have also resulted in habitat fragmentation in its regular breeding grounds (Hill and Lloyd, 2017)
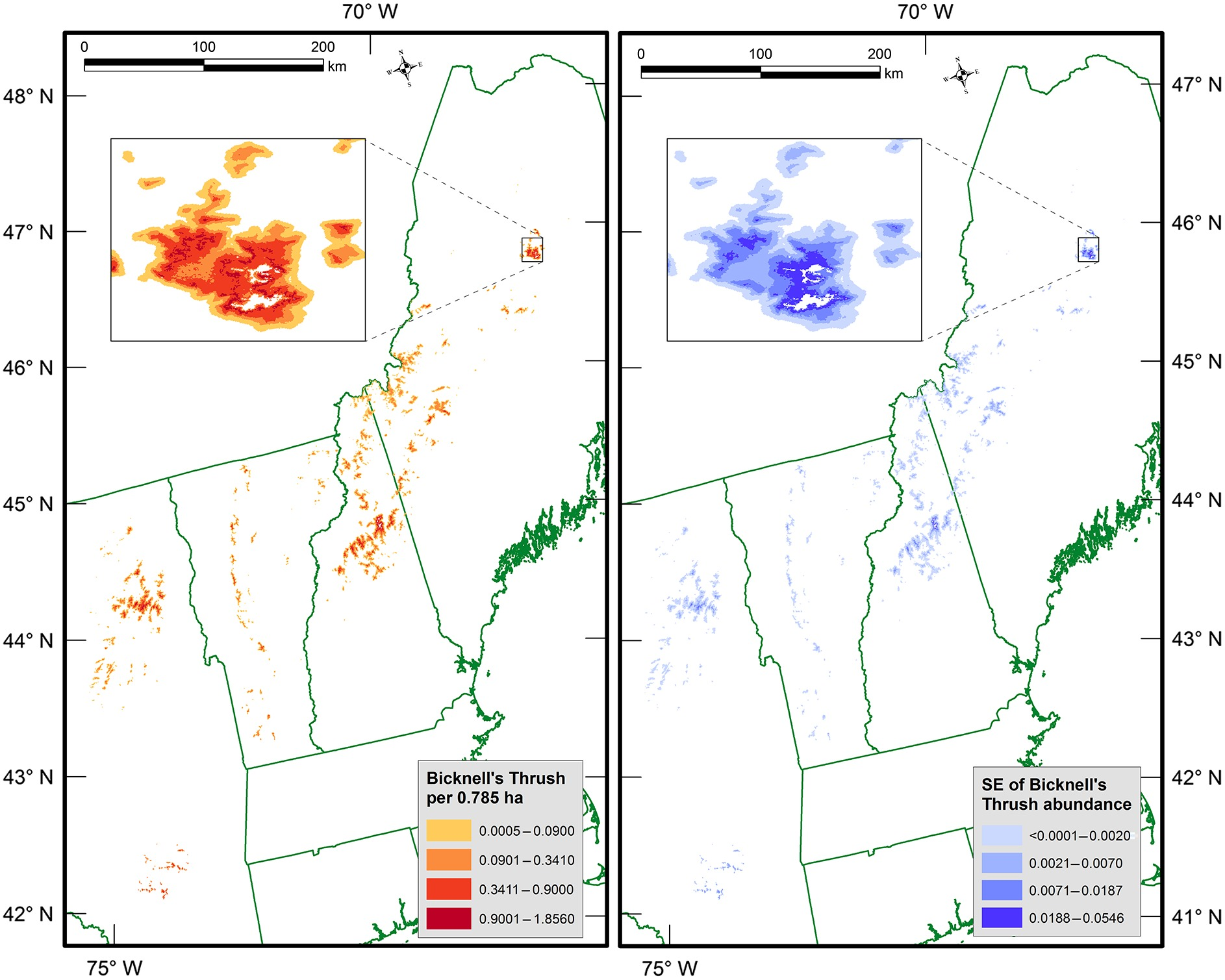
Wetland habitats are some of the best environments for water birds, as they provide shelter for breeding, nesting, and rearing of young, along with easy access to water and food. Many birds have adapted to these habitats and are reliant on the quality of the wetlands, such as the grebes. Vermont’s primary wetland habitat is the Lake Champlain Basin, encompassing more than 300,000 acres of wetlands and home to a diverse population of waterfowl (USGS Wetlands as Bird Habitat). Wetland habitats for birds are being impacted by Harmful Algal Blooms and the effects of warming waters on freshwater plants and animals that birds depend on.
4.4.4 Notable Species in Vermont
The National Audubon Society conducted a comprehensive study of future range suitability for North American bird species. The full study can be accessed in an interactive online format via their Survival By Degrees website. For Vermont, under a Representative Concentration Pathway (RCP) 8.5 Climate Scenario with an overall increase of 3.0°C, 94 bird species were identified as moderately to highly vulnerable to climate change, resulting in loss of suitable habitat ranges. Here, we highlight four bird species because of their importance in Vermont and potential climate-related declines: hermit thrush, golden-winged warbler, common loon, American bittern, and pied-billed grebe.
Hermit Thrush
The state bird of Vermont, the hermit thrush (Catharus guttatus) is a small migratory bird that winters in the US. With a wide geographic range, current climate projections predict an overall 73 percent loss in current summer range by 2080, along with a large-scale shift northwards (Glennon et al., 2019). The hermit thrush’s unique overwintering range makes it more vulnerable to shifts in winter seasonality.
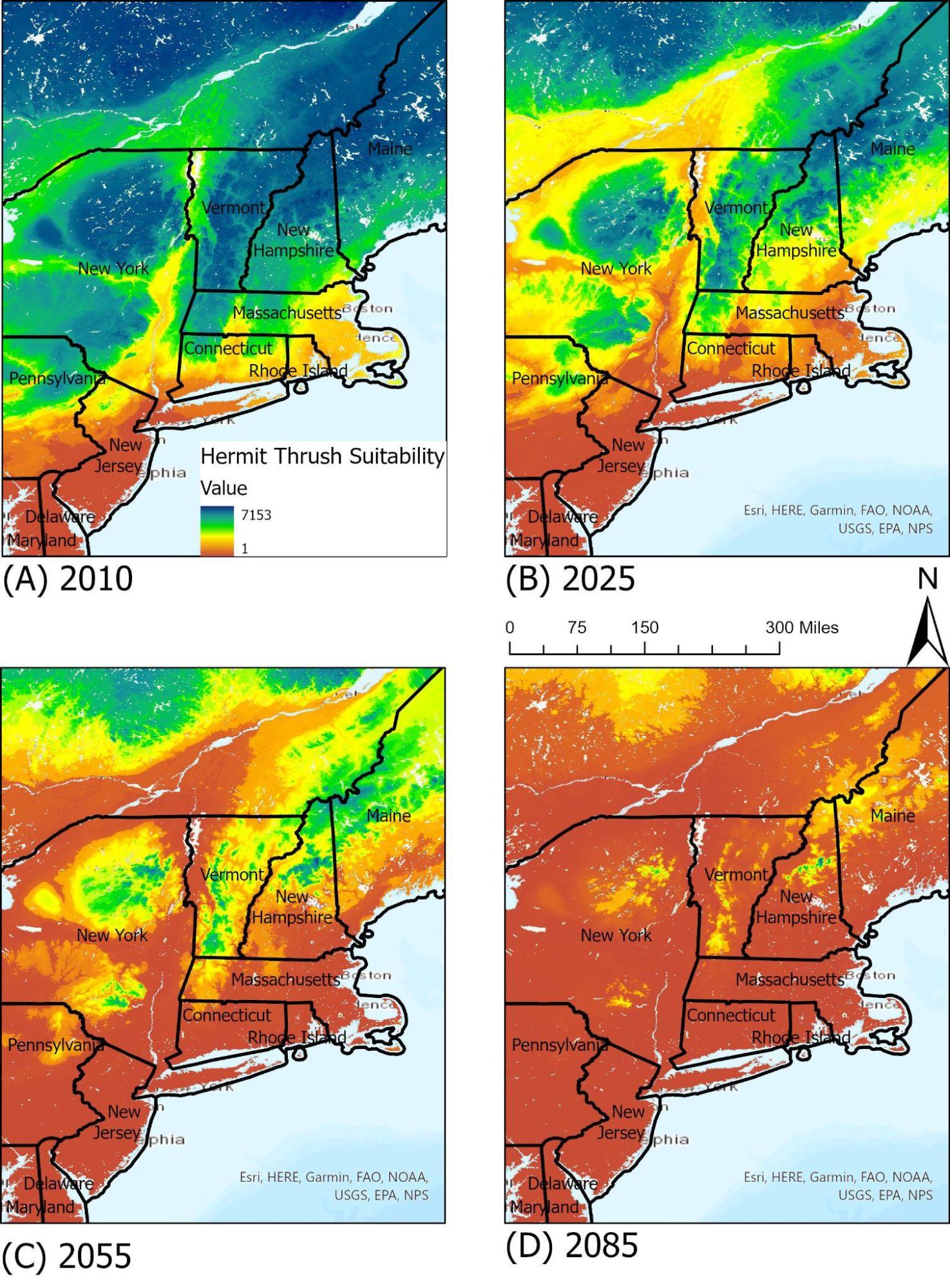
Golden-Winged Warbler
The golden-winged warbler (Vermivora chrysoptera) is an interesting bird as an early successional species, requiring habitats with shrubs, sparse trees, and grass understories (see Climate Change in Forests chapter). As a result of increased human development and successive stages towards more forested areas, many of its ranges have been lost, and populations have dwindled. Currently, Vermont is the only New England state that hosts a population of golden-winged warblers (REF Audubon Center). Due to its specific habitat requirements, the golden-winged warbler can find some suitable habitats within Vermont at this point, but it is predicted that by 2055 much of the suitable ranges in the northeast will shift northwards (Figure 4-7)
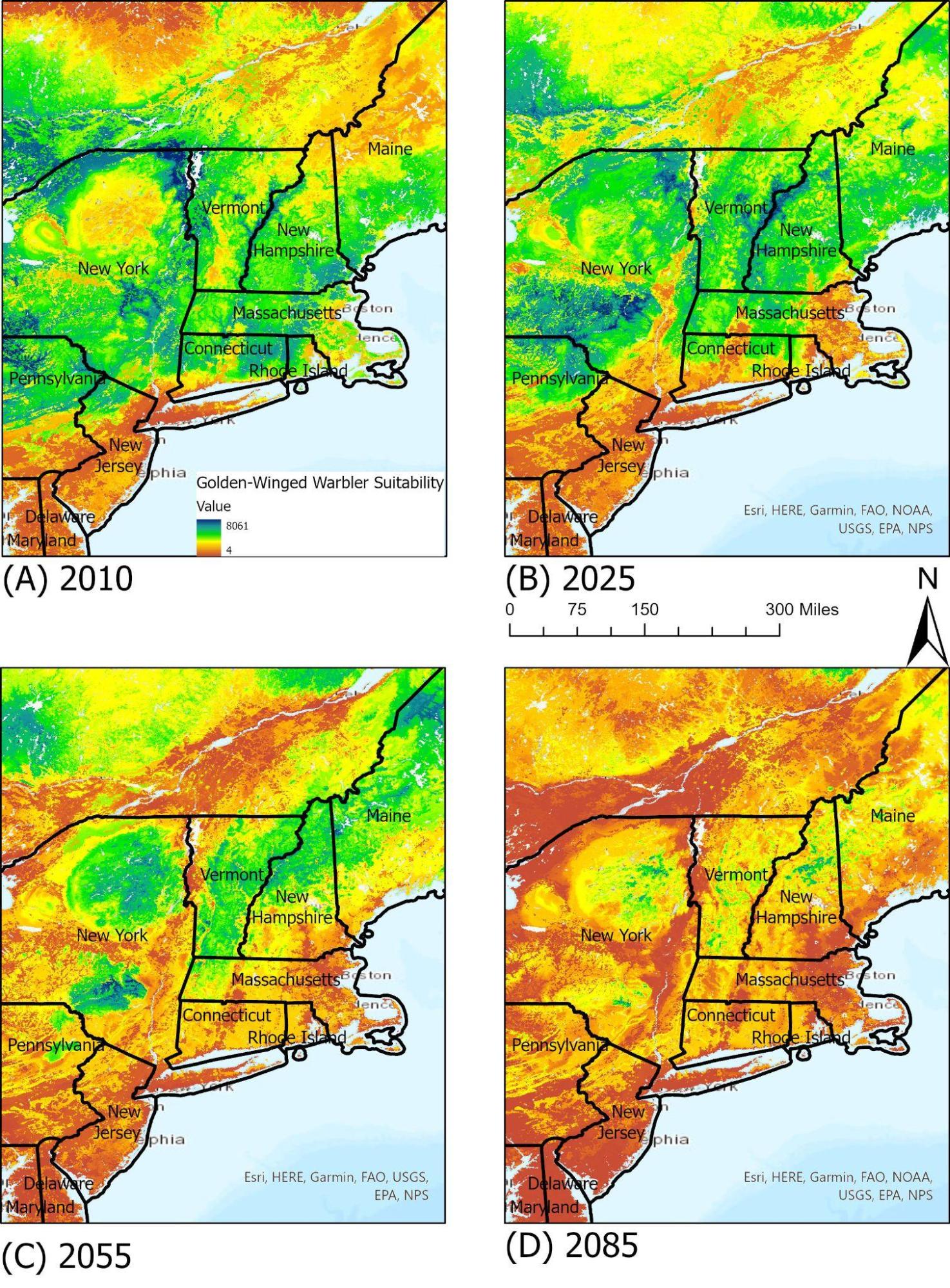
Common Loon
As one of four species of loon in North America, and the only breeding species in Vermont, the common loon (Gavia immer) is an iconic species in Vermont. It is often used as an indicator for lake health due to its reliance on available lake habitats and water levels. It shares much of its territory with humans, as nests will often be built along the shoreline of lakes and ponds during the nesting seasons, and so it is particularly susceptible to human interference and to water quality. Bianchini et al. (2020) found that anthropogenic effects on water bodies can have negative effects on reproductive success and population declines in the common loon, and cites acid rain and lake acidity as one of the key stressors. This demonstrates the intersectionality between wildlife and their environment, and highlights the importance of maintaining water quality and pH levels for wildlife health. Today, the common loon does have suitable ranges in Vermont’s lakes and ponds, but under RCP 8.5 may have very few suitable areas by 2050 due to a combination of water quality and annual temperature changes.
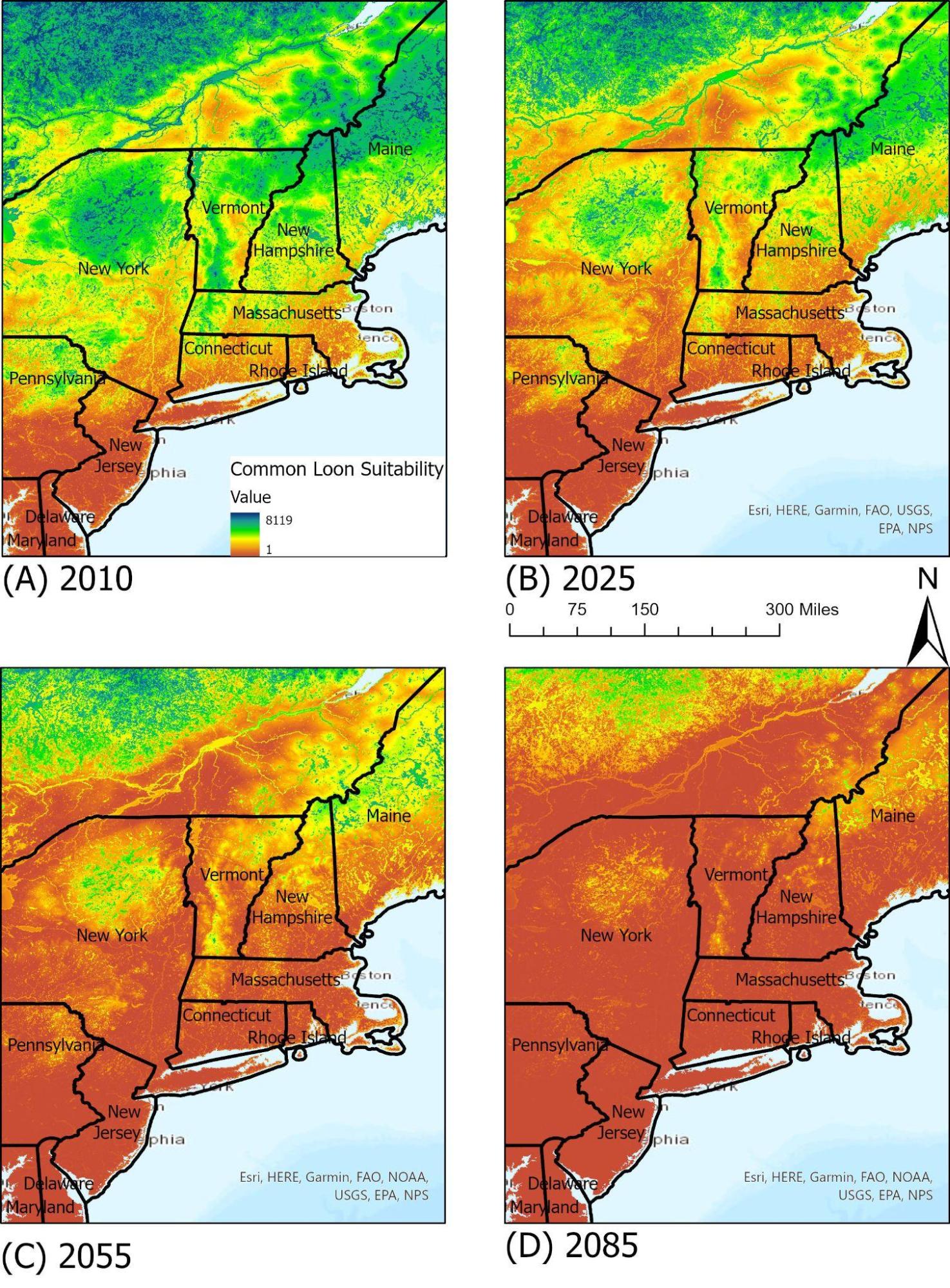
American Bittern
A medium-sized, solitary heron, the American bittern is a common sight in freshwater marshes and wetlands, often hunting for fish and aquatic life in the shallow water. Its main nesting and foraging grounds are marshes and reedy lakes during both summer and winter, and occasionally it may feed in dry grass fields. On a national scale, the American bittern has experienced serious declines in the Southern portions of its breeding ranges, largely due to loss of available habitats and its reliance on large marshes. In Vermont, the American bittern has its suitable ranges located near water bodies such as lakes and ponds, much like the common loon. By 2085, under the RCP 8.5 scenario, its available ranges in the New England area will see an overall shift northwards as annual temperatures and seasonality shifts (Figure 4-9).
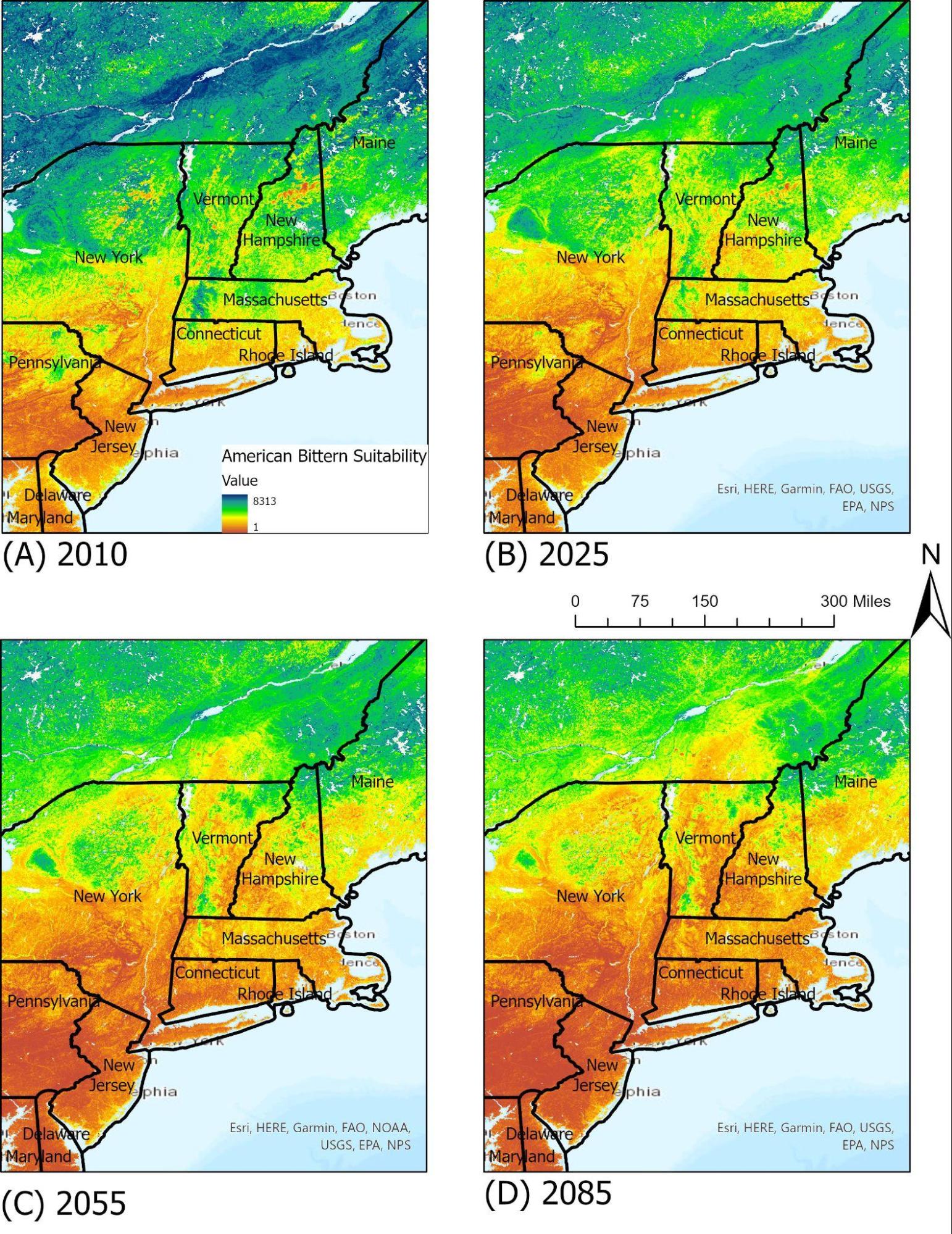
Pied-Billed Grebe
The pied-billed grebe is a common bird species found in temperate climates in North America in ponds, lakes, and marshes. Their diet consists of insects, fish, and other aquatic life. As they are reliant on water bodies and appropriate marsh habitats, pied-billed grebe in Vermont are most often seen around Lake Champlain and similar water bodies. Under the RCP 8.5 climate scenario, available suitable habitat for the pied-billed grebe is expected to decrease over time due to a variety of factors, including invasive aquatic plants and degradation of existing aquatic ecosystems. By 2085, they may be largely limited to lowland areas and the Champlain Valley of Vermont (Figure 4-10).
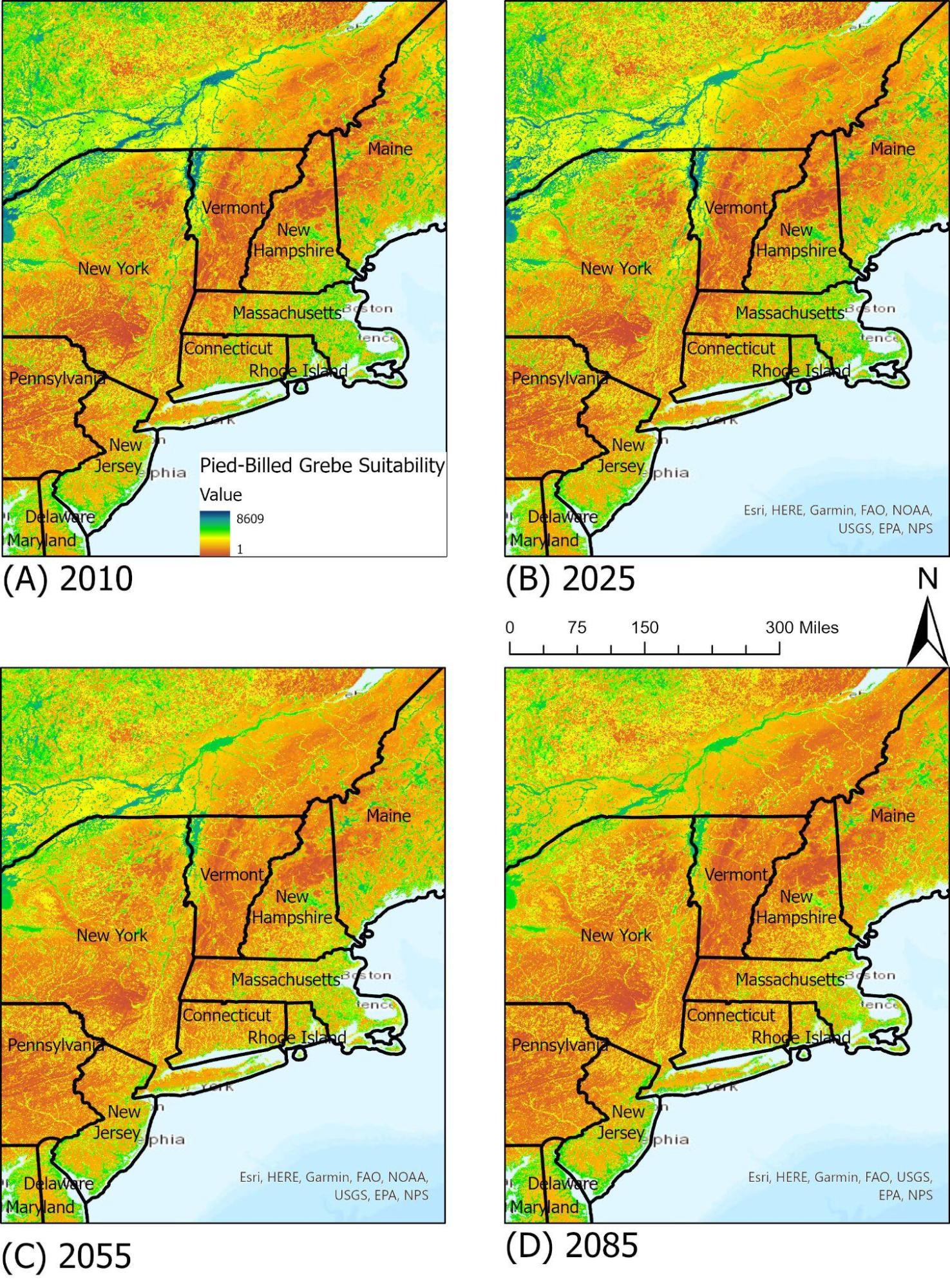
4.5 Bats
Like birds, many bat species are aerial insectivores and face similar challenges due to climate change. Vermont is currently home to 9 species of bats, with 3 of them being migratory tree bats that travel to the Southern US, Central America, and South America for winter. Like birds, phenological timing during migration and reproductive timing is critical to their success. There have been studies done across the United States that attempt to study climate and weather impacts on bat migrations (Luncan et al. 2013), which can provide insight as to their conditions in Vermont. Studies done on the Indiana bat (Myotis sodalis) have found that warming temperatures have had a prominent effect on habitat suitability, and increased warming may lead to potential range shifts and large-scale movement patterns northward, with the potential of new bat species roosting in Vermont (Loeb and Winters, 2013).
The main issue that bats face in Vermont at this point in time is the fallout from the arrival of white-nose syndrome in 2006, which has resulted in the loss of more than 5.7 million bats in the Northeastern United States. The two most common species in Vermont, the little brown bat (Myotis lucifugus) and the northern-long-eared bat (Myotis septentrionalis), have seen a 90% decline in population. Commonly contracted due to exposure to a fungus inside bat hibernacula (Pseudogymnoacscus destructans), this disease results in attacks on the bare skin of hibernating bats and can cause abnormal behavior that can result in early mortality. With such drastic declines, Vermont is losing out on a number of critical services supplied by bats, and an important component of the food web is also missing.
It is uncertain how the impacts of climate change will interact with that of climate change. While microclimate conditions have been shown to affect the growth of the fungus, it is difficult to predict how climate change and the white-nose syndrome epidemic will interact on a large scale. Further research is required to understand the effect that rises in temperature and variation in cave microclimate (humidity) will have on bat infection rates and mortality (Maher et al, 2012).
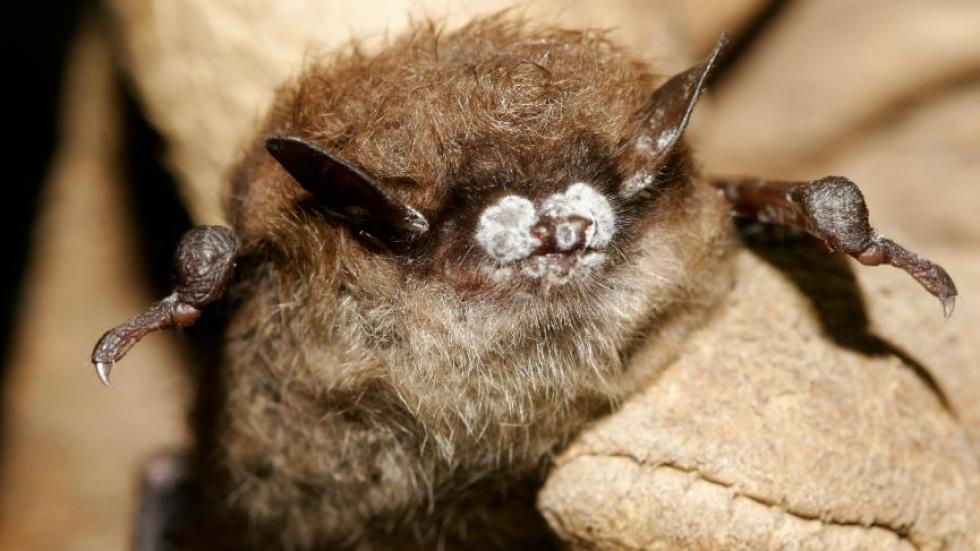
Bats provide critical ecosystem services like pollination, fruit dispersal, and arthropod control. Due to their sensitivity to environmental stressors, they can also serve as ecological indicators of habitat quality. With insectivorous bats occupying high trophic levels, monitoring of their populations and health would allow researchers to identify contaminants or environmental disturbances very easily. Dietary accumulation increases as you go up the food chain, therefore bats would likely be able to show the consequences of pollutants in the environment much sooner than herbivorous insects or birds (Jones et al., 2013). To prevent disease outbreaks like white-nose syndrome from occurring, wildlife managers and researchers should be encouraged to collect baseline population data for a variety of species, not just bats, so that negative stressors and impacts in a population can be quickly discovered and investigated.
4.6 Large Ungulates (Moose and Deer)
Though moose (Alces alces) and white-tailed deer (Odocoileus Virginianus) are both large ungulates, their responses to climate change serve as direct points of contrast rather than comparison. Moose are generally anticipated to become physiologically stressed in response to warming temperature trends and deer have clear survival advantages due to the warmer temperature and decreased snowpack. So, while not in direct competition, their population trends do follow opposing directions in response to warming climate patterns.
White-tailed deer distribution and abundance are heavily affected by winter severity, which is often measured by two variables, ambient temperature and snow depth (high confidence). Winter snow depth can affect body conditions of deer and increase movement costs, primarily affecting maternal body condition and reducing future reproductive success. Deer are often reported to have a thermal tolerance close to 19.4°F (-7°C), below which begins the potential for physiological and behavioral responses. Average annual winter temperatures are trending to be greater than this thermal tolerance and extremes are changing—extremely cold days (max. temperature 50°F) are increasing (see Climate Change in Vermont chapter). More variation within the winter season could bring unexpected physiological or behavior changes (e.g., shedding, foraging). Snow depth affects deer but can be a problematic measurement, as snow drifts skew measurements at particular locations or times and can lead to inconsistencies. Snowfall, while not a perfect proxy for snow depth, is a more accurate measure of snow, and has been decreasing for the last 60 years in Vermont (see Climate Change in Vermont chapter) but is greater than when measurements began in the 1930s. As such, the near-term outlook is that snow, and therefore snow depth, may not change substantially in the coming decades but could be seriously reduced beyond 50 years in the future. These winter conditions can directly affect deer populations through survival effects, as well as indirectly by impacting reproduction and fecundity of females (Weiskopf et al., 2019).
Substantial evidence has been collected to indicate that increasing temperature conditions and decreased winter severity leads to increased abundance of white-tailed deer; changes in migratory behavior; altered foraging, habitat selection, or behavior; and a potential increase in hemorrhagic disease outbreaks (Weiskopf et al., 2019). There are complexities to this situation that should be considered. One example is the potential loss of hemlock trees, an important thermal shelter for deer, due to warming winters and the invasion of the HWA. These changes to forest composition could result in near-term difficulties for deer during this shifting temperature regime (McClure, 1990).
Range shifts in deer may occur due to warming temperatures and as a result of climate-induced habitat changes in the northern U.S. For example, oak species (Quercus spp.) are predicted to increase in both range and abundance in some areas of Northern Wisconsin and Upper Michigan, providing increased mast production that serves as high-energy food sources for deer. This could encourage a steady shift in deer population northwards into previously unsuitable habitats; however, deer can also browse oak heavily enough to limit the survival of regenerating stands. There may be a cyclical dynamic between deer and oak abundance as oak species try to shift northward.
In Vermont, hunting has always been a major component of human interaction with the landscape. Annual harvests are tracked by the Vermont Fish and Wildlife Department. Deer harvests across Vermont have been steadily increasing in the more northern region of Vermont in the past few decades (Figure 4-11), although this could reflect either a change in population or in hunter effort. Figures 4-12 and 4-13 represent mean deer harvests by town per decade in a southern and northern county, respectively, illustrating an overarching pattern where deer harvests are observed in increasing numbers northwards over time. Wildlife managers should take these shifts into account when developing landscape management plans, as well as the predicted boom in deer population as ambient winter temperature increases per year. Increased hunting interest or success rates and higher bag limits are major reasons for these observed spikes in harvest, which serve an important role in controlling the population of deer and moose.
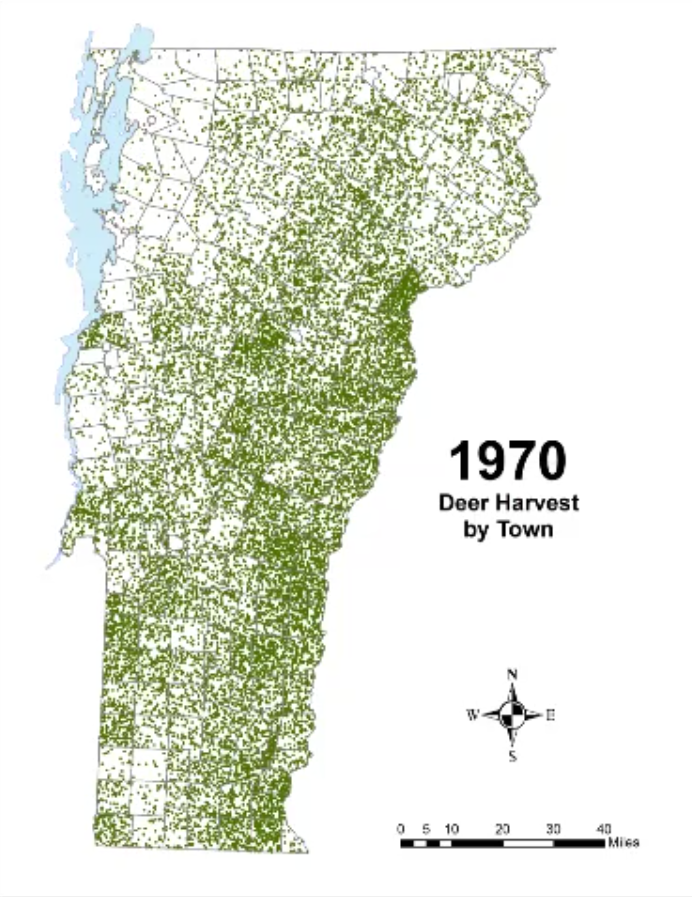
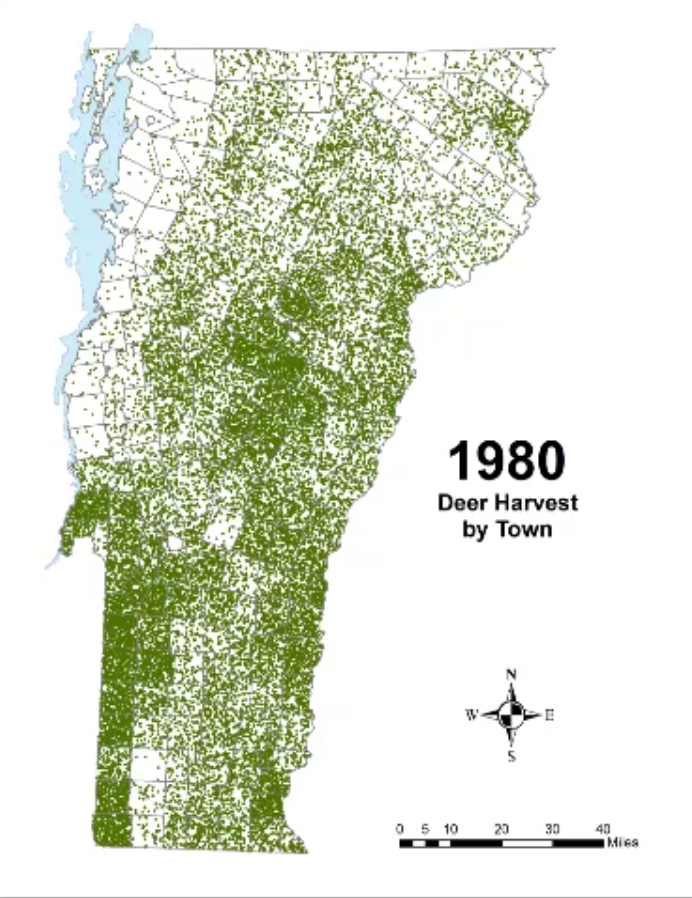
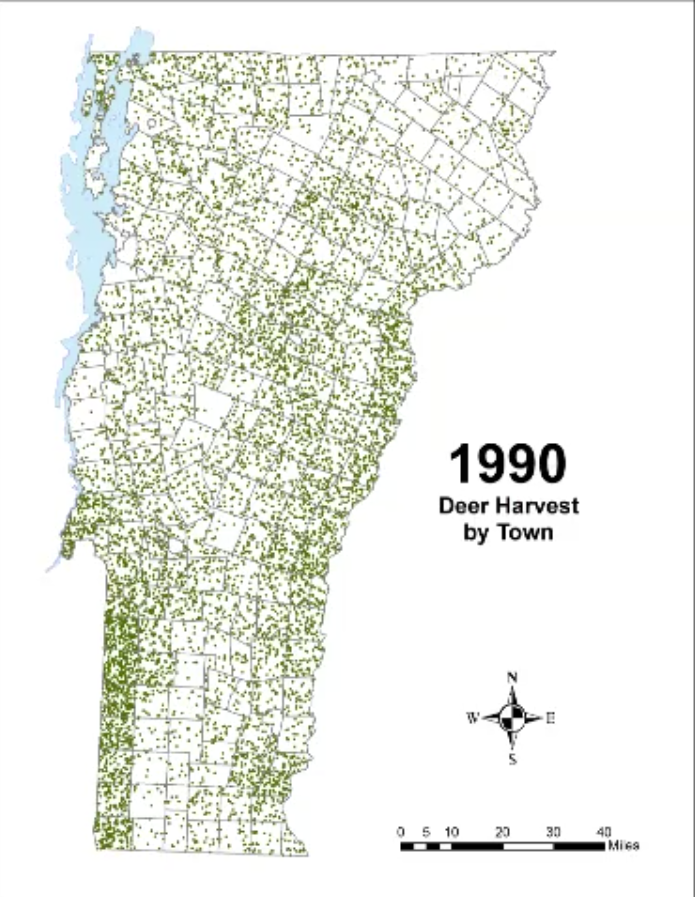
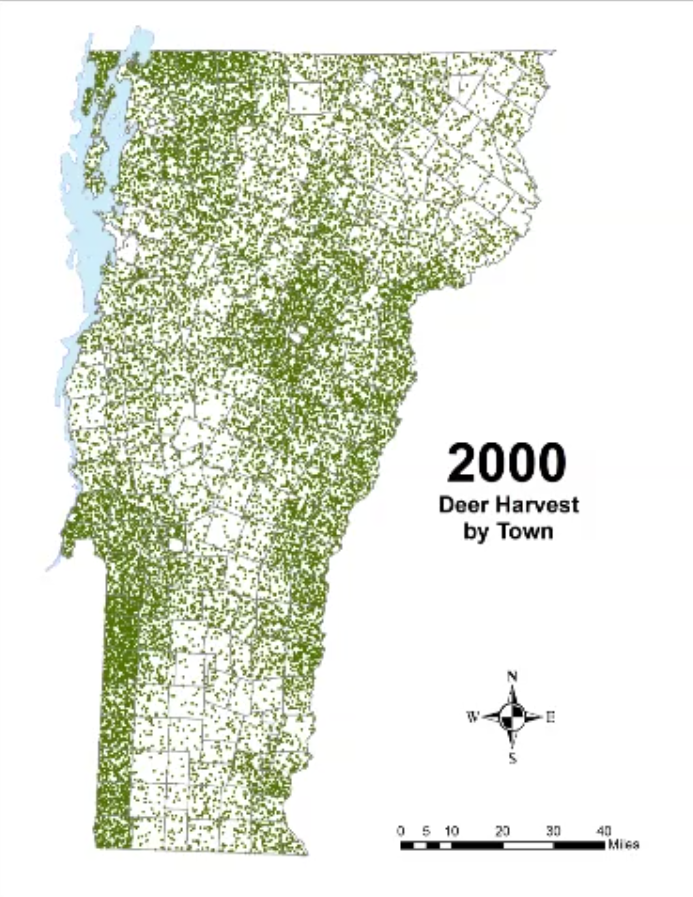
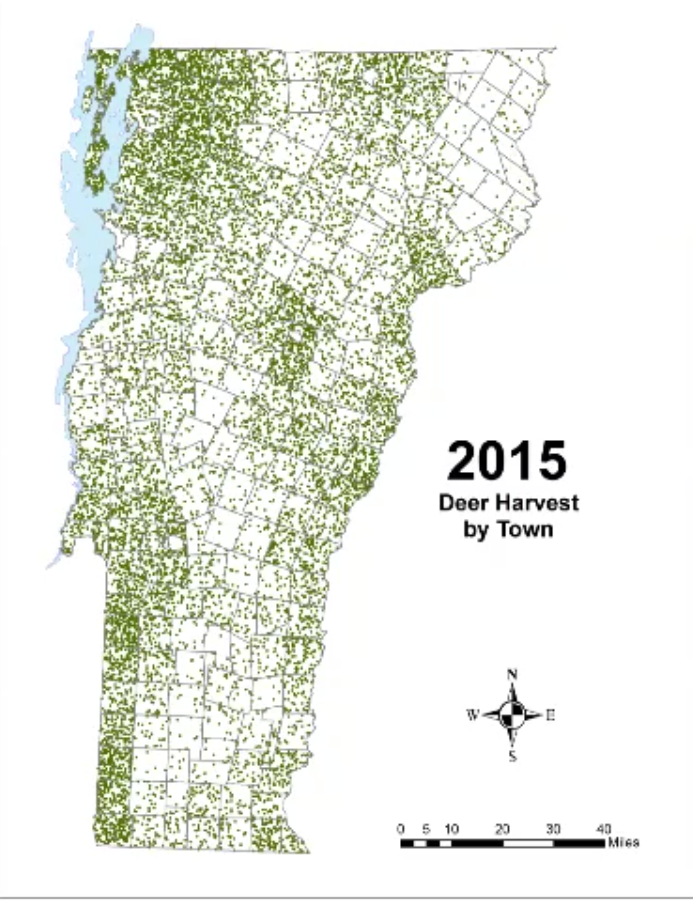
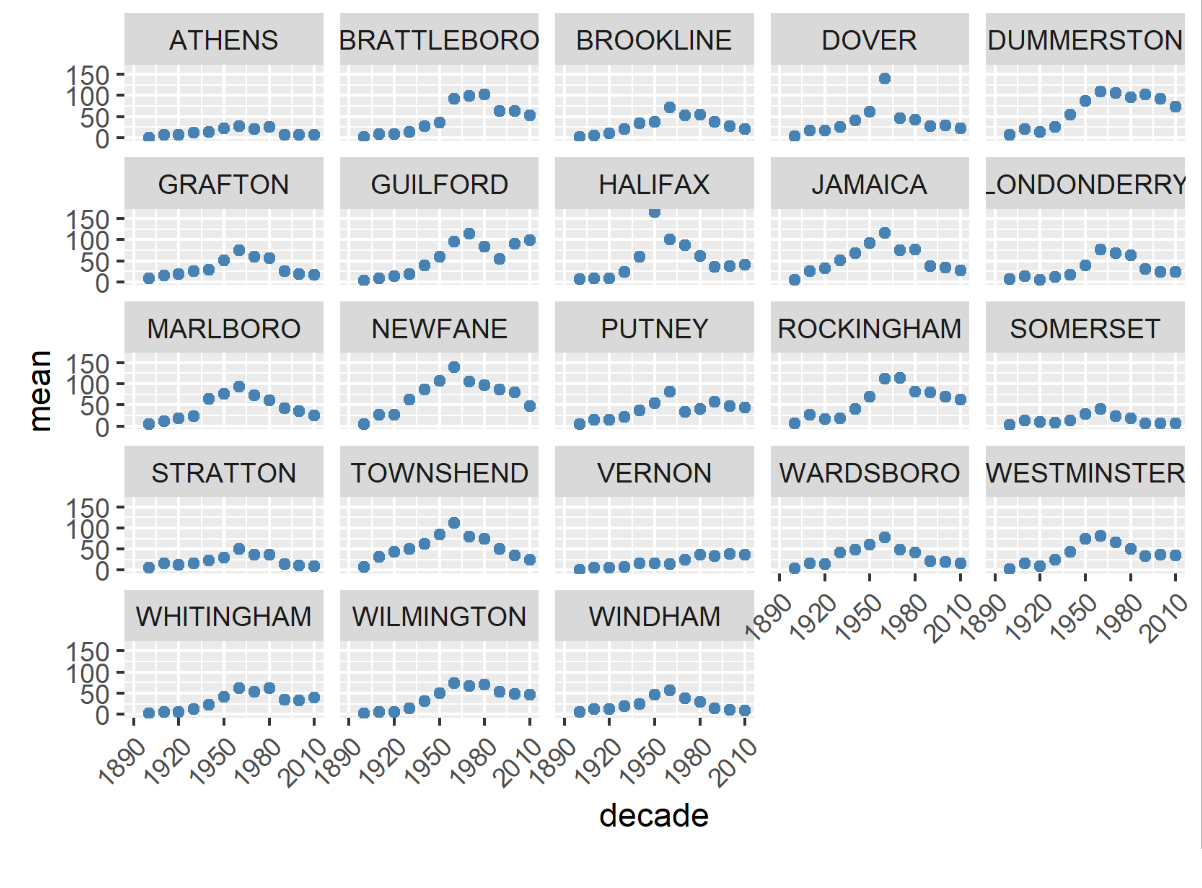
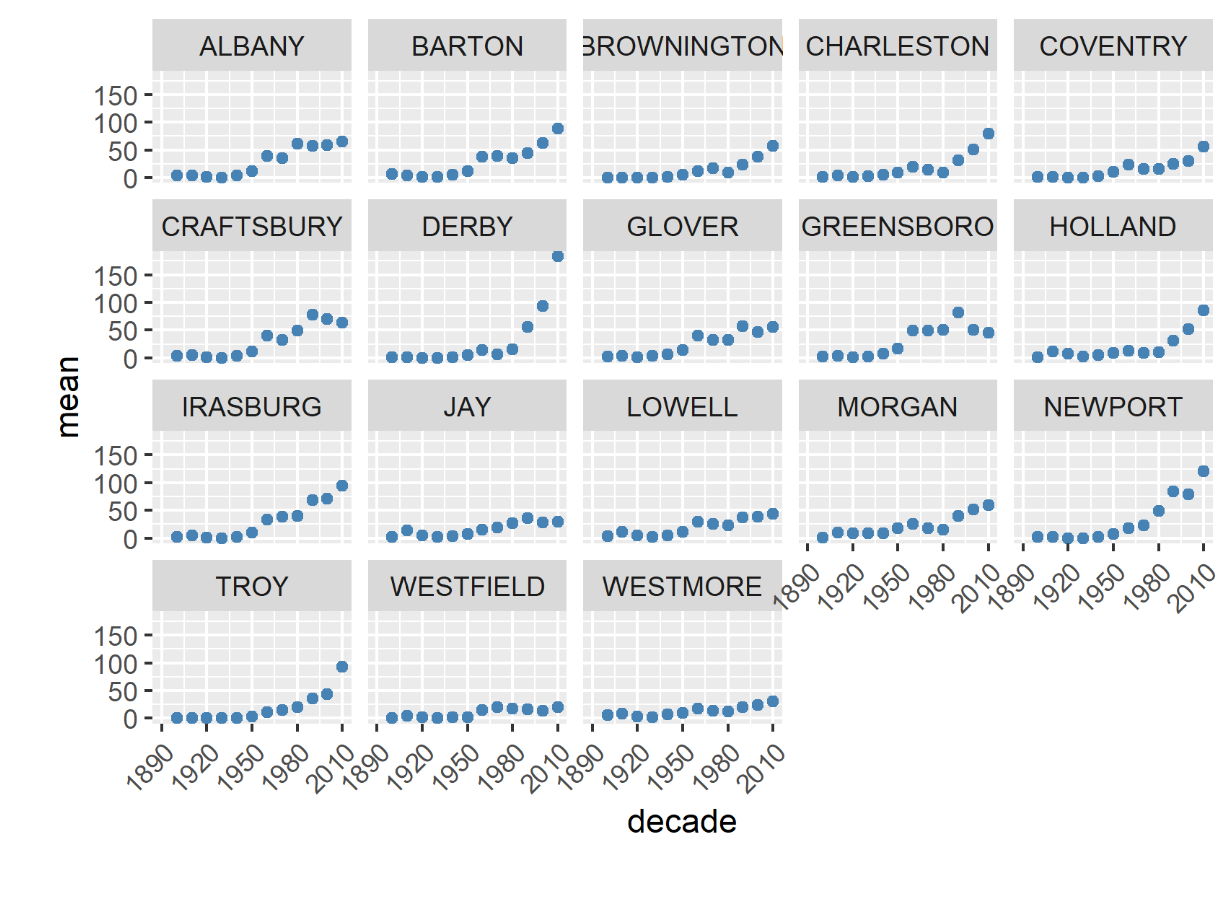
CASE STUDY: Deer Hunting as a Social-Ecological Climate Challenge
Objective and Approach
Deer hunting may be the most recognizable expression of Vermont’s hunting culture (Boglioli, 2009), making it a useful case to investigate possible ecological and social impacts of climate change on hunting in the Green Mountain State. This subsection uses a nexus approach, which is helpful for looking at data and trends from many fields (Liu et al., 2018). Here, we examine the outlook for deer hunting in Vermont under climate change through the lenses of wildlife population health and social demand, supported by links to three other chapters of the Vermont Climate Assessment. This analysis integrates a high-confidence ecological assessment with two less-certain social scenarios to highlight risks and opportunities for wildlife management.
BOX 1: KEY MESSAGES
Ecological conditions under climate change will favor increased deer populations in Vermont causing undesirable damages to forests (high confidence), but the social prognosis for hunting as a culturally important practice for recreation, food, and deer management is uncertain (medium confidence).
Ecological and Social Assessments
Accounting for climate change, the ecological prognosis in Vermont for white-tailed deer, a widely-sought game species, is promising (VT Fish and Wildlife Department 2020). Vermont’s deer population is broadly healthy, with substantial local variation that requires active management to balance. Even-aged forests provide limited habitat in the more remote portions of the state and support fewer deer than historical precedent, while some agricultural areas attract an overabundance of deer resulting in competition that reduces individual fitness and leads to overbrowse in regenerating forests (VT Fish and Wildlife Department 2020). In the coming centuries, forest community structure is likely to shift towards transitional hardwood species like red oak and shagbark hickory (see Climate Change in Forests chapter), providing more abundant forage for deer (Rodenhouse et al., 2009; Blossey et al., 2019). Shorter term changes in forest structure, such as the continued decline of eastern hemlock by the end of the century (see Climate Change in Forests chapter), may introduce stress if not immediately congruent with decreased winter intensity, but may also result in increased early successional habitat that favors deer (Fortin, personal communication, 2021). Potential urban and peri-urban development (see Community Development chapter) is unlikely to pose a threat to the species, which is relatively tolerant of development (Gaughan and Destefano, 2005
Anticipating that deer populations in Vermont will grow in the coming decades, the ecological need for increased deer harvest to maintain healthy population sizes, limit overbrowse of regenerating forests, and minimize human-deer conflict will also likely rise (VT Fish and Wildlife Department, 2020). It is less certain if the social demand for hunting will match the ecological need for harvest. Key to this balance are several concurrent factors that, while not directly linked to climate change, may affect the social demand, or tolerance, for deer hunting in Vermont. Nationally, an aging participant base, urbanization, and changing wildlife values are associated with declining per-capita hunter participation since the mid twentieth century (Manfredo et al., 2020; Winkler and Warnke, 2013). Vermont exhibits each of these trends, albeit with anecdotal evidence of less severe declines in demand for, and tolerance of, hunting. Hunting license sales have fallen from over 100,000 in the early 1980s to just over 50,000 in 2016. However, sales increased during the pandemic—even controlling for new data collected by the state on multi-year license holders (Figure 4-14). Likewise, while a plurality of Vermonters hold “mutualist” wildlife values not commonly associated with support for hunting (Figure 4-15 for trend and definition), public support for deer hunting in Vermont remains high (VT Fish and Wildlife Department, 2020; Boglioli, 2009).
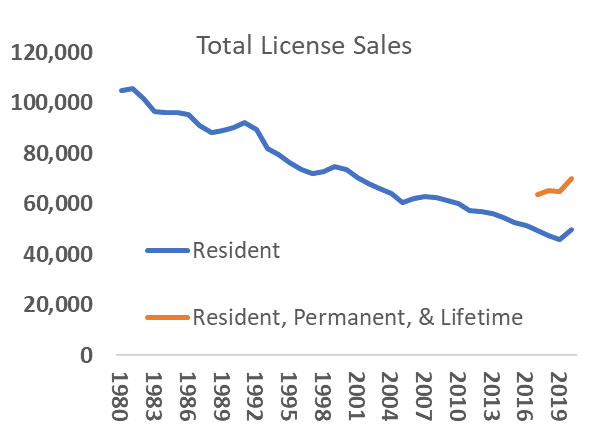
Urbanization also affects hunter participation. Urban and suburban development may increase due to Vermont’s recent trends in parcelization (Fidel and McCarthy, 2018), and desirability to non-residents for second homes and outdoor amenities (See Community Development). Changing demographics and land uses could fuel further value change in the Vermont population. Well-established research on wildlife values suggests that urbanization correlates with value shifts away from the “traditionalist” values held by a plurality of Vermont hunters (Dietsch et al., 2018; Manfredo et al., 2020). However, measures of public values and attitudes around wildlife that represent more diverse traditions than those shown in Figure 4-16 are gaining traction in research and practice (e.g. Himes and Muraca, 2018). Future wildlife management in Vermont that recognizes diverse values and attitudes will be especially important for honoring the longstanding wildlife practices—including deer hunting—of the Abenaki (Wiseman, 2001 p. 42-43; 56). In addition, alongside increased land development, there is a current trend of decreasing public access to private lands, rigorously documented in Eastern states (Jagnow et al., 2006; Snyder et al., 2008; Walberg et al., 2018) but only anecdotally described in Vermont to date. Increased limits on public access to private land would further challenge hunting and other outdoor pursuits (see Recreation and Tourism chapter). In a worst-case scenario, hunter decline and decreased land access, driven by social value change and shifting land-ownership patterns and norms, would result in hunting becoming a less effective approach to balancing Vermont’s growing deer population—creating a social and ecological challenge for wildlife managers.
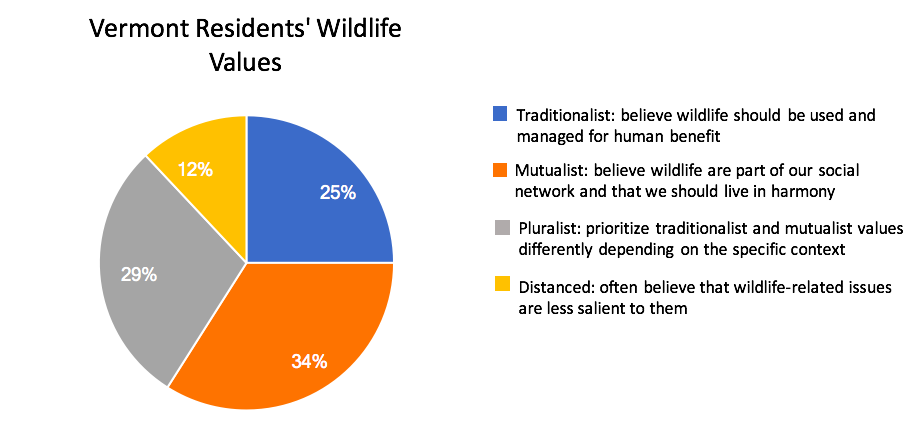
Alternatively, recent trends suggest a different plausible future scenario rooted in the widely-documented potential of major social and ecological upheavals to reshape human relationships with the environment and natural resources (Marshall et al., 2005; Tidball, 2012). The COVID-19 pandemic drove an increase in hunting participation, as both license sales and enrollment in hunter education courses (now available online) exceeded benchmarks from recent years (Figure 4-14; Meier, personal communication, 2021). Recent research into the pandemic’s impacts on outdoor activities and values from nature confirms these recent trends, suggesting that Vermonters are relying on nature to cope with crises (Morse et al., 2020)—and that the pandemic may have created new opportunities for outdoor experiences like hunting (see Box 1). While the pandemic is an imperfect proxy for the sustained social changes brought by climate change, it does illustrate the possibility of resurgent social demand for hunting, consistent with the literature of nature engagement in response to upheaval (Tidball, 2012). Maintaining this momentum as the pandemic recedes, and nurturing it in the face of future upheavals, will require a multi-pronged effort tapping into both traditional and emerging veins of public support for, and interest in, hunting. The Vermont Land Trust’s efforts to include access for hunting on conservation easements and Vermont Fish and Wildlife’s “Wild Kitchen” programming, which connects diverse hunting and locavore communities, are examples of two such initiatives (VT Fish and Wildlife Department, 2021a; Slayton, 2019). Emerging research suggests pathways to augment this work. Future efforts could engage with rapidly emerging affinity groups in the hunting community—through organizations like Hunters of Color, Artemis Sportswomen, and Backcountry Hunters and Anglers—and university students to connect new hunters with land and resources and could continue to tie into Vermont’s strong local food ethic to appeal to these stakeholders (Vayer et al., 2021). With proactive engagement, a best-case scenario could match a growing deer population with sustained or increased social demand for hunting.
BOX 2: New Hunters, the Pandemic, and Cultural Relevance
“I grew up accompanying my dad hunting occasionally, but lost that connection when he died when I was twenty. I have been interested for a number of years, especially thinking about connection to land and the animals we eat, but felt there were just enough barriers to keep me from getting (re)started. This past fall was when I first started hunting in earnest. Thinking about it, a number of things coincided at the right time to make it possible for me to get over the hump. Crossbows being allowed for all hunters, hunters safety courses being online, having access to land, having lots of time because of the pandemic, and having friends to help me get through the myriad questions one needs answered to just get started. Certainly, I don’t think I would be hunting if not for the pandemic. I ended up spending a good part of last year in a rural place and with lots of time on my hands, and so was able to give foraging, fishing, and hunting the many hundreds of hours needed to start. During deer season in October and November, I felt I was able to catch up on the many hours of ‘five years hunting with a dad’ that some hunters are lucky to receive.”
– Kristian Brevik (33), Burlington, Vermont
Synthesis
This case study finds potential to meet the ecological challenge of an expanding deer herd under climate change with increased hunter participation. However, this best case outcome is not set in stone. Active effort will likely be required to support and sustain any new social demand for hunting that arises in response to future crises. Absent a proactive approach to both the ecological challenges facing—and social dimension affecting—Vermont’s deer herd and hunting culture, a misalignment between deer population and social demand for hunting could occur if value change and shifting patterns of land ownership and access are exacerbated by climate change. With this in mind, sustaining deer hunting in Vermont during an era of climate change will require collaborative engagement from ecologists, social scientists, community planners, and a wide variety of stakeholders.
4.7 Aquatic Species
As global mean temperature continues to increase annually, subsequent warming of water bodies will also have a profound impact on the ecosystem. As aquatic species are exposed to new thermal habitats, community structures and trait distributions may see radical reshuffling as organisms respond to warming climates.
4.7.1 Riverine and Stream Ecosystems
Warming climate trends will have a variety of effects on physical aspects of riverine systems, with major consequences to organisms living in the system (Ficke et al., 2007). Increased water temperature reduces the amount of available oxygen in the system, potentially leading to the creation of hypoxic/anoxic zones where there is very little oxygen in the water, often causing health issues and mortality in aquatic species living there. Common fish species such as trout and salmon, and warm-water fish like smallmouth bass rely on groundwater discharges for cooler refuges during summer seasons. These refugia will decrease in availability as groundwater temperature is expected to increase over time (Ficke et al., 2007). Rivers will also see changes to the timing and amount of precipitation seen throughout the year. Warming temperatures will likely lead to reduced snowpack—decreasing spring flows and negatively affecting fish species. Increased temperature may also lead to increased toxicity of pollutants in the system, as common pollutants such as heavy metals and organophosphates become more toxic with rising temperatures.
Within riverine systems, many species of freshwater fish are expected to migrate due to shifts in water temperature. These migrations will result in compositional shifts and loss of ecosystem services, as freshwater fish communities shift from cold-water species to more warm-water species. Fluctuations in ambient water temperature may also result in shifts in seasonal flow and potential changes to growth rates of aquatic species (Xu et al., 2010). Biswas et al. found that freshwater lake communities in Ontario, Canada, are predicted to see an increase in species richness of 60-81% due to this migration, but a subsequent decline in functional diversity. Jones et al. also made similar predictions, finding that in the US on a national level, current climate projections and increased air temperature will lead to increased water temperature, rendering some fish habitats unsuitable for native species. As cold-water fish species such as trout are more susceptible to changes in thermal habitats, the loss of available cold-water fish habitats may result in considerable economic loss. This would have dramatic impacts on the present distribution of sport and commercially valuable fish and cause considerable losses in commercial and recreational fisheries.
Fish Passage and Ecological Connectivity
Similar to terrestrial animals, fish are heavily reliant on their physical landscape and connectivity in order to migrate, move through different environments at different life stages, and take advantage of multiple habitat types. Habitat fragmentation is a key issue that has widespread impacts on fish, such as salmonids like the Eastern brook trout, and overall aquatic diversity (Hudy et al., 2008). Infrastructure such as roads and dams have severely hampered the mobility of aquatic species, and current road-stream crossing designs have proven ineffective in most cases. Heller (2007) has demonstrated that in many water bodies of the US, over half to two thirds of current road-stream crossings serve as a form of barrier for fish migrating and for fish seeking cold refuge during hot spells. During flooding and storm events, road-stream crossings and culverts are particularly susceptible to damming as the floodwater may exceed capacity and clog it with debris and sediment. Climate change is already affecting not only water temperatures but also frequency of abnormal weather events such as heavy rainfall events (see Climate Change in Vermont chapter) and increased stream-flows (see Water Resources chapter). As Vermont’s annual precipitation has been increasing by 7.5 inches since the 1900s, precipitation across all seasons is expected to increase and put strain on the infrastructure, creating more issues for wildlife expected to utilize them (see Climate Change in Vermont chapter).
Brook Trout
Brook trout (Salvelinus fontinalis), Vermont’s state fish, are an important component of Vermont’s aquatic fisheries—they make up a large portion of Vermont’s cold-water fishery resources, along with brown and rainbow trout. Together these three species are the most popular fish for open water anglers (Responsive Management 2021); although Vermont anglers have reported less time is spent on brook trout fishing than in previous decades, declining from 79% of anglers in 1990, to 67% in 2010 and a statistically significant decrease to 58% in 2020 (Responsive Management 2021).
Brook trout provide a clear example of how climate change may affect aquatic species as a whole. Like many other fish, they are known to be stenothermal, and are unable to tolerate anything beyond a small range of temperatures. As such, they require cold streams (often below 20°C) for their optimal habitat. Due to global temperature increases, brook trout are expected to experience range contractions, severely limiting their ability to reproduce and inhabit stream ecosystems. Based on current warming scenarios, a warming of 3.8°C is predicted to result in a reduction of 89% to thermally-suitable habitats for brook trout in North Carolina and other Southeastern portions of North America. In Southern Ontario, Canada, a study of two headwater streams found that a 4.1°C increase in summer air temperature could reduce thermally suitable habitats by 42% and 30% respectively (Meisner, 1990).
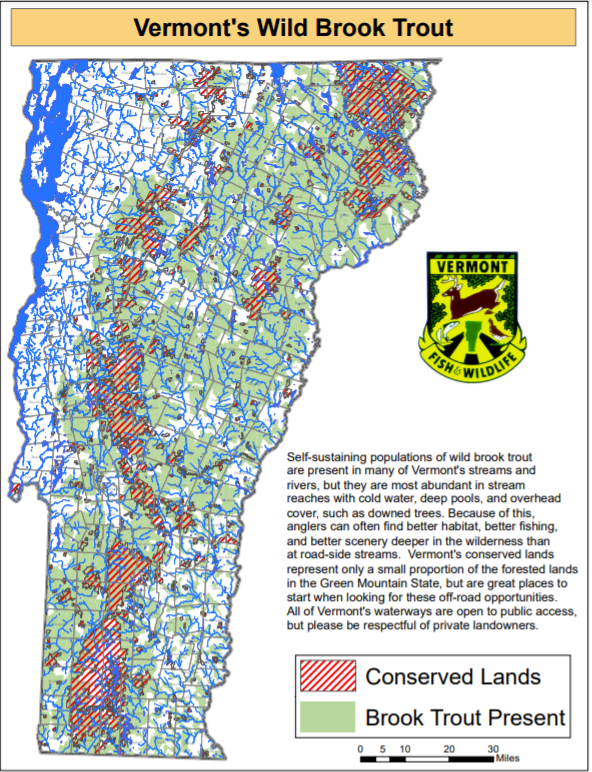
4.7.2 Lake Systems
Climate Change impacts on algal blooms (HABs)
Lake ecosystems are an attractive environment for both humans and wildlife, with many of Vermont’s lakes serving important biological functions for nesting birds and aquatic species that live there, as well as offering recreational and economic benefits for humans. The projected changes to water and air temperature raise concerns about the general health of the lake ecosystem and possible consequences that wildlife and landscape managers should be aware of.
One of the main climate change concerns is eutrophication and the health of water bodies in Vermont. Eutrophication is the process where water bodies become heavily enriched with nutrients (e.g., nitrogen, phosphorus) that feed plant and algae material in the water. The nutrients typically come from human activities, such as excess urban and agricultural runoff. The enrichment of nutrients causes increased macrophyte and algal growth. Increases in macrophytes can stimulate algal blooms that lay on the water surface, blocking sunlight and limiting the growth potential of underwater plants while also being a nuisance for many lake-goers. Once these algal blooms die, they are decomposed by bacteria that use up the available dissolved oxygen within the water. This leads to more hypoxic and anoxic zones where dissolved oxygen levels are so low and unsuitable for fish habitability that it can even lead to mortality (with large incidents of mortality known as fish kills). In temperate lakes, this shift towards eutrophic systems can often result in the extirpation of economically important species such as salmonids in favor of small species like cyprinids and gizzard shad. (Jones et al., 2013). These have little to no economic value from a fisheries perspective, and would want to be avoided at all cost by fishery managers.
Climate change is predicted to play a larger role over time as increased temperatures will result in lower stream flows—increasing residence times of water and reducing the flushing of nutrients from the water, which increases trophic statuses. This uptick in nutrients and minerals often leads to the presence of algal blooms; these blooms are not only a visible nuisance for humans, but can also result in harmful ecological effects.
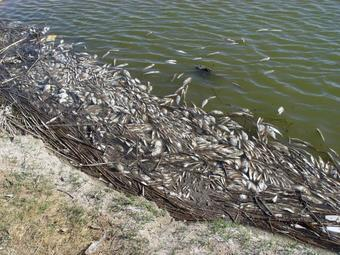
4.7.3 Vernal Pools and Amphibians
Scattered across Vermont’s forested landscape are thousands of vernal pools—small, isolated depressions that fill with spring rains and snow melt, but dry by late summer. Created by glaciation more than 10,000 years ago during the Pleistocene era, these ephemeral wetlands provide critical breeding habitat for several amphibians and support an abundant and diverse invertebrate community, many of which would become locally extirpated without vernal pools. Although seemingly small and insignificant at first glance, vernal pools are keystone ecosystems that significantly influence the surrounding forest by “exporting” large amounts of biomass (in the form of post-metamorphic amphibians) from the aquatic system to the terrestrial environment. However, climate change could have profound effects on vernal pool functions, the impacts of which would undoubtedly extend into the surrounding landscape.
Hydroperiod (the length of time a pool contains water) is the most important abiotic factor affecting the composition and productivity of vernal pool-dependent wildlife (Semlitsch et al., 1996). Annual variation in hydroperiod is directly related to year-to-year changes in weather patterns, especially precipitation, as well as pool size and depth (Winter et al., 2001). A pool must hold water for at least three months following ice-out in order to support successful breeding of wood frog (Lithobates sylvaticus) and four months to support successful breeding of mole salamanders (Ambystoma spp.) (Brooks, 2004). At the same time, a pool must dry occasionally to exclude fish populations that could predate on these larval amphibians that evolved in fishless environments. Under current climate change scenarios of earlier and stronger evapotranspiration during the growing season, combined with frequent droughts and shifts in the timing of precipitation, it is likely that many pools will dry earlier and remain dry longer, resulting in an increase in the frequency of pool-breeding amphibian reproductive failures. While these species are well-adapted to a “boom-bust” cycle of recruitment, where partial or complete reproductive failures are offset by large cohorts in “boom” years, an increase in the frequency of “bust” years could limit the viability of many populations.
In Vermont, the majority (90%) of pools studied were less than ¼-acre in size and shallower than 2-feet in depth (Faccio et al., 2013). These small, shallow, abundant pools would be disproportionately affected by climate-induced changes to hydroperiod, resulting in the increased isolation of the larger, remaining pools that could serve as hydrologic refugia (Cartwright et al. 2021). Such isolation would affect the ability of juvenile amphibians to disperse between pools, impact genetic connectivity of populations, and limit the recolonization of pools where breeding populations have been extirpated—all of which are critical to the long-term viability of metapopulations.
Pool-breeding salamanders in the genus Ambystoma, including spotted, Jefferson, and blue-spotted, are fossorial outside of the brief egg-laying period in spring. Although it would seem that spending most of their lives underground would buffer them from the effects of climate change, a recent study in Ontario showed that body condition of spotted salamanders declined over a 12-year monitoring period (2008–2019) due, at least in-part, to increased summer and autumn temperatures (Moldowan et al. 2021).
Additionally, a reduction in snowpack could lead to increased winter mortality of several species of frogs, including wood frog, spring peeper (Pseudacris crucifer), and grey treefrog (Hyla versicolor). All three species hibernate just below the leaf litter on the forest floor. As the temperature drops below freezing, they flood their bloodstream with glucose—which serves as an antifreeze to protect their cells from damage, even while the frogs freeze solid and their hearts stop beating. However, the glucose only provides protection down to about 20°F, so they are completely reliant on an insulating blanket of snow to keep them alive on frigid winter nights (O’Connor and Rittenhouse, 2016).
Building Resilience
From a landscape perspective, Vermont is particularly focused on maintaining ecological functions and connectivity within its forests and waters, with many conservation biologists and landscape ecologists agreeing that development of a resilient ecosystem network can alleviate the effects of habitat fragmentation and climate change. In the face of rapid climate change, populations of species will likely shift their geographic ranges to landscapes with the least amount of habitat fragmentation. In order to develop a more resilient network, landscape management strategies such as development of wildlife corridors and riparian zones near water bodies are currently being maintained and introduced to Vermont’s ecosystems (Interview with Robert Zaino of VT Fish and Wildlife Department-Interviewed by George Ni, 11/3/2020).
4.8 Traceable Accounts
Traceable accounts describe the confidence level—the degree of certainty in the scientific evidence—for each key message resulting from this chapter. This analysis is based on the U.S. Global Change Research Program guidance in the Fourth National Climate Assessment (USGCRP, 2018).
Confidence Level
- Very High – Strong evidence (established theory, multiple sources, confident results, well-documented and accepted methods, etc.), high consensus
- High – Moderate evidence (several courses, some consistency, methods vary, and/or documentation limited, etc.), medium consensus
- Medium – Suggestive evidence (a few sources, limited consistency, models incomplete, methods emerging, etc.), competing schools of thought
- Low – Inconclusive evidence (limited sources, extrapolations, inconsistent findings, poor documentation and/or methods not tested, etc.), disagreement or lack of opinions among experts
Key Message 1
As climate change worsens, 92 bird species of Vermont, including the iconic common loon and hermit thrush, are expected to disappear from the landscape within the next 25 years.
- Description of evidence base: Evidence for climate change projections and shifts to bird species’ ranges were developed by the National Audubon Society for use in Audubon’s report Survival by Degrees: 389 Bird Species on the Brink.
- Major uncertainties: Possibly missing/not considering all of the possible predictors and variables that might affect bird species distributions, especially specific bird species or geographic traits.
- Description of confidence and likelihood: There is very high confidence that shifts in global temperature and seasonality will have dramatic shifts to Vermont’s bird species, resulting in extirpation and extinction of many species.
Key Message 2
Increasing warming trends are expected to result in an increase in white-tailed deer population and a mirrored decrease in moose population, which may have long-term impacts on Vermont’s forest composition. Managing social systems (e.g. hunting) to account for changing public tolerance and demand for deer may provide one avenue to minimize this risk if undertaken proactively.
- Description of evidence base: Annual harvest data of moose and deer hunting in Vermont was obtained from Vermont’s Fish and Wildlife Department, with records spanning from 1900-2019. Multiple studies across northern latitudes indicate favorable conditions for white-tailed deer under climate change (Rodenhouse et al., 2009; Gaughan and Destefano, 2005). Nationwide and state-specific trends show declines in hunting participation since 1980 (VT Fish and Wildlife Department, 2021b). However, public support for deer hunting in Vermont remains high (Boglioli, 2009; VT Fish and Wildlife Department, 2020).
- Major uncertainties: Harvest data alone is not necessarily an accurate depiction/substitute for demographic data. There are also implicit biases to the data (Harvest data implies a human-centric bias to the data as the occurrences/records are only the ones that were actually hunted by humans, so actual numbers are sure to vary).
- Description of confidence and likelihood: While the harvest data alone does not give sufficient evidence of climate change’s impacts on ungulate populations, studies done across North America have found sufficient evidence that white-tailed deer and moose populations have opposite reactions to common warming trends, and these dynamics have long-term impacts to forest composition and structure. As of right now there is medium confidence that white-tailed deer and moose populations have been affected until appropriate population data has been gathered. Sufficient hunting participation and public support for hunting are key if the practice is to remain a method for managing Vermont’s deer population.
Key Message 3
As warming trends reduce the severity of winters, the subsequent warming waters will have adverse effects on lake and river systems, including increased risk for harmful algal blooms (HABs) and reduced overall biodiversity and health in lake ecosystems.
- Description of evidence base: Evidence of warming lake temperatures come from numerous studies on Lake Champlain and other water bodies that have been conducted on climate change’s impacts on aquatic ecosystems and limnology (Smetzer, 2012; Kaushal, 2012).
- Major uncertainties: Trends and annual water temperatures of Vermont’s lakes and rivers are consistent and monitored through both government agencies and citizen science efforts. The full impact of these trends on overall aquatic health is unclear, as the creation of HABs is only partially affected by the annual water temperature.
- Description of confidence and likelihood: There is high confidence that warming trends will result in overall increases in summer surface water temperatures, as well as increased precipitation and runoff events. According to the available research on HABs, it is possible that HABs may increase in frequency and intensity due to the flushing of nutrients into Vermont’s waterways as well as increases in water temperature.
4.9 References
Adams, R., & Hayes, M. (2021). The Importance of Water Availability to Bats: Climate Warming and Increasing Global Aridity (pp. 105–120). https://doi.org/10.1007/978-3-030-54727-1_7
Austin, B. C., & McKinley, D. (n.d.). Benefits of Stream Simulation Designed Road-Stream Crossings beyond Aquatic Organism Passage. 29.
Austin, J. (n.d.). Fish and Wildlife Conservation and Climate Change Adaptation in Vermont. 7.
Baldigo, B. P., Lawrence, G. B., Bode, R. W., Simonin, H. A., Roy, K. M., & Smith, A. J. (2009). Impacts of acidification on macroinvertebrate communities in streams of the western Adirondack Mountains, New York, USA. In Ecological Indicators (Vol. 9, Issue 2, p. 14). https://doi.org/10.1016/j.ecolind.2008.04.004
Bartomeus, I., Ascher, J. S., Wagner, D., Danforth, B. N., Colla, S., Kornbluth, S., & Winfree, R. (2011). Climate-associated phenological advances in bee pollinators and bee-pollinated plants. Proceedings of the National Academy of Sciences, 108(51), 20645–20649. https://doi.org/10.1073/pnas.1115559108
Bartomeus, I., Park, M. G., Gibbs, J., Danforth, B. N., Lakso, A. N., & Winfree, R. (2013). Biodiversity ensures plant-pollinator phenological synchrony against climate change. Ecology Letters, 16(11), 1331–1338. https://doi.org/10.1111/ele.12170
Bateman, B. L., Wilsey, C., Taylor, L., Wu, J., LeBaron, G. S., & Langham, G. (2020). North American birds require mitigation and adaptation to reduce vulnerability to climate change. Conservation Science and Practice, 2(8), e242. https://doi.org/10.1111/csp2.242
Bee_Basics_North_American_Bee_ID.pdf. (n.d.). Retrieved July 7, 2021, from https://efotg.sc.egov.usda.gov/references/public/SC/Bee_Basics_North_American_Bee_ID.pdf
Betts, A. K. (2011). Vermont Climate Change Indicators. Weather, Climate, and Society, 3(2), 106–115. https://doi.org/10.1175/2011WCAS1096.1
Bianchini, K., Tozer, D. C., Alvo, R., Bhavsar, S. P., & Mallory, M. (2020). Drivers of declines in common loon (Gavia immer) productivity in Ontario, Canada. The Science of the Total Environment. https://doi.org/10.1016/j.scitotenv.2020.139724
Biswas, S. R., Vogt, R. J., & Sharma, S. (2017). Projected compositional shifts and loss of ecosystem services in freshwater fish communities under climate change scenarios. Hydrobiologia, 799(1), 135–149. https://doi.org/10.1007/s10750-017-3208-1
Block, K., Molyneaux, R., Gibbs, L., Alkemade, N., Baker, E., MacDougall, C., Ireton, G., & Forbes, D. (2019). The role of the natural environment in disaster recovery: “We live here because we love the bush.” Health & Place, 57, 61–69. https://doi.org/10.1016/j.healthplace.2019.03.007
Blossey, B., Curtis, P., Boulanger, J., & Dávalos, A. (2019). Red oak seedlings as indicators of deer browse pressure: Gauging the outcome of different white-tailed deer management approaches. Ecology and Evolution, 9(23), 13085–13103. https://doi.org/10.1002/ece3.5729
Boglioli, M. (2009). A Matter of Life and Death: Hunting in Contemporary Vermont. Univ of Massachusetts Press.
Brittain, J. E. (2008). Mayflies, Biodiversity and Climate Change. In F. R.Hauer & A. Welch (Eds.), International Advances in the Ecology, Zoogeography, and Systematics of Mayflies and Stoneflies (pp. 1–14). University of California Press. https://doi.org/10.1525/california/9780520098688.003.0001
Brook-Trout-Conserved-Lands-Map.pdf. (n.d.). Retrieved July 29, 2021, from https://vtfishandwildlife.com/sites/fishandwildlife/files/documents/Fish/Brook-Trout-Conserved-Lands-Map.pdf
Bumble bee ( Bombus ) distribution and diversity in Vermont, USA: a century of change | SpringerLink. (n.d.). Retrieved July 7, 2021, from https://link.springer.com/article/10.1007%2Fs10841-018-0113-5
Cadieux, P., Boulanger, Y., Cyr, D., Taylor, A. R., Price, D. T., & Tremblay, J. A. (2019). Spatially explicit climate change projections for the recovery planning of threatened species: The Bicknell’s Thrush (Catharus Bicknelli) as a case study. Global Ecology and Conservation, 17, e00530. https://doi.org/10.1016/j.gecco.2019.e00530
Cartwright, J., T.L. Morelli, and E.H. Campbell Grant. 2021. Identifying climate-resistant vernal pools: hydrologic refugia for amphibian reproduction under droughts and climate change. Ecohydrology https://doi.org/10.1002/eco.2354
Chen, I.-C., Hill, J. K., Ohlemüller, R., Roy, D. B., & Thomas, C. D. (2011). Rapid Range Shifts of Species Associated with High Levels of Climate Warming. Science, 333(6045), 1024–1026. https://doi.org/10.1126/science.1206432
Cooke, B. J., & Carroll, A. L. (2017). Predicting the risk of mountain pine beetle spread to eastern pine forests: Considering uncertainty in uncertain times. Forest Ecology and Management, 396, 11–25. https://doi.org/10.1016/j.foreco.2017.04.008
Covino, K. M., Horton, K. G., & Morris, S. R. (2020). Seasonally specific changes in migration phenology across 50 years in the Black-throated Blue Warbler. The Auk, 137(ukz080). https://doi.org/10.1093/auk/ukz080
Dawe, K. L., & Boutin, S. (2016). Climate change is the primary driver of white-tailed deer (Odocoileus virginianus) range expansion at the northern extent of its range; land use is secondary. Ecology and Evolution, 6(18), 6435–6451. https://doi.org/10.1002/ece3.2316
Dennis, D. F. (1993). An Empirical Study of Posting Private Nonindustrial Forests. Wildlife Society Bulletin (1973-2006), 21(1), 6–10.
Diefenbach, D. R., Rathbun, S. L., Vreeland, J. K., Grove, D., & Kanapaux, W. J. (2016). Evidence for Range Contraction of Snowshoe Hare in Pennsylvania. Northeastern Naturalist, 23(2), 229–248. https://doi.org/10.1656/045.023.0205
Dietsch, A. M., A. W. Don Carlos, M. J. Manfredo, T. L. Teel, and L. Sullivan. 2018. Vermont State Report. America’s Wildlife Values, Colorado State University, Department of Human Dimensions of Natural Resources, Fort Collins, CO.
Ellis, D., & Vokoun, J. C. (2009). Earlier Spring Warming of Coastal Streams and Implications for Alewife Migration Timing. North American Journal of Fisheries Management, 29(6), 1584–1589. https://doi.org/10.1577/M08-181.1
Faccio, S.D., M. Lew-Smith, and A. Worthley. 2013. Vermont Vernal Pool Mapping Project, 2009 – 2012. Final Report to the Vermont Fish and Wildlife Natural Heritage Information Project. Vermont Center for Ecostudies, Norwich, VT. 40 pages https://val.vtecostudies.org/projects/vermont-vernal-pool-atlas/vermont-vernal-pool-atlas-results/.
of the Vermont Department of Fish and Wildlife
Faccio, S.D., J.D. Lambert, and J.D. Lloyd. 2017. The status of Vermont forest birds: A quarter century of monitoring. Vermont Center for Ecostudies, Norwich, VT. 32 pages https://vtecostudies.org/projects/forests/vermont-forest-bird-monitoring-program/results/.
Ficke, A. D., Myrick, C. A., & Hansen, L. J. (2007). Potential impacts of global climate change on freshwater fisheries. Reviews in Fish Biology and Fisheries, 17(4), 581–613. https://doi.org/10.1007/s11160-007-9059-5
Frick, W. F., Stepanian, P. M., Kelly, J. F., Howard, K. W., Kuster, C. M., Kunz, T. H., & Chilson, P. B. (2012). Climate and Weather Impact Timing of Emergence of Bats. PLOS ONE, 7(8), e42737. https://doi.org/10.1371/journal.pone.0042737
Garroway, C. J., & Broders, H. (2005). The quantitative effects of population density and winter weather on the body condition of white- tailed deer (Odocoileus virginianus) in Nova Scotia, Canada. https://doi.org/10.1139/Z05-118
Gaughan, C. R., & Destefano, S. (2005). Collaboration for community-based wildlife management. Urban Ecosystems, 8(2), 191. https://doi.org/10.1007/s11252-005-3265-5
GCB.Townsend.pdf. (n.d.). Retrieved March 15, 2021, from http://canuck.dnr.cornell.edu/research/pubs/pdf/GCB.Townsend.pdf
Geluso, K., Lemen, C., Freeman, P., Andersen, B., White, J., & Johnson, H. (2019). Variation in Reproductive Timing for the Northern Long-Eared Myotis (Myotis septentrionalis) Across Nebraska. Western North American Naturalist, 79, 581. https://doi.org/10.3398/064.079.0411
Geographic distribution of plant pathogens in response to climate change—Shaw—2011—Plant Pathology—Wiley Online Library. (n.d.). Retrieved February 27, 2021, from https://bsppjournals.onlinelibrary.wiley.com/doi/10.1111/j.1365-3059.2010.02407.x
Gérard, M., Vanderplanck, M., Wood, T., & Michez, D. (2020). Global warming and plant–pollinator mismatches. Emerging Topics in Life Sciences, 4(1), 77–86. https://doi.org/10.1042/ETLS20190139
Gillespie, N., Unthank, A., Campbell, L., Anderson, P., Gubernick, R., Weinhold, M., Cenderelli, D., Austin, B., McKinley, D., Wells, S., Rowan, J., Orvis, C., Hudy, M., Bowden, A., Singler, A., Fretz, E., Levine, J., & Kirn, R. (2014). Flood Effects on Road–Stream Crossing Infrastructure: Economic and Ecological Benefits of Stream Simulation Designs. Fisheries, 39(2), 62–76. https://doi.org/10.1080/03632415.2013.874527
Glennon, M. J., Langdon, S. F., Rubenstein, M. A., & Cross, M. S. (2019). Temporal changes in avian community composition in lowland conifer habitats at the southern edge of the boreal zone in the Adirondack Park, NY. PLOS ONE, 14(8), e0220927. https://doi.org/10.1371/journal.pone.0220927
Hamilton, A. T. (2010). Vulnerability of biological metrics and multimetric indices to effects of climate change. Freshwater Science, 29(4), 1379–1396. https://doi.org/10.1899/10-053.1
Hayhoe, K., Wake, C. P., Huntington, T. G., Luo, L., Schwartz, M. D., Sheffield, J., Wood, E., Anderson, B., Bradbury, J., DeGaetano, A., Troy, T. J., & Wolfe, D. (2007). Past and future changes in climate and hydrological indicators in the US Northeast. In Climate Dynamics (Vol. 28, Issue 4, p. 27). https://doi.org/10.1007/s00382-006-0187-8
Hill, J. M., & Lloyd, J. D. (2017). A fine-scale U.S. population estimate of a montane spruce–fir bird species of conservation concern. Ecosphere, 8(8), e01921. https://doi.org/10.1002/ecs2.1921
Himes, A., & Muraca, B. (2018). Relational values: The key to pluralistic valuation of ecosystem services. Current Opinion in Environmental Sustainability, 35, 1–7. https://doi.org/10.1016/j.cosust.2018.09.005
Huang, M., Ding, L., Wang, J., Ding, C., & Tao, J. (2021). The impacts of climate change on fish growth: A summary of conducted studies and current knowledge. Ecological Indicators, 121, 106976. https://doi.org/10.1016/j.ecolind.2020.106976
Hudy, M., Hanson, T., Gillespie, N., & Smith, E. (2008). Distribution, Status, and Land Use Characteristics of Subwatersheds within the Native Range of Brook Trout in the Eastern United States. North American Journal of Fisheries Management, 28, 1069–1085. https://doi.org/10.1577/M07-017.1
Hurlbert, A. H., & Liang, Z. (2012). Spatiotemporal Variation in Avian Migration Phenology: Citizen Science Reveals Effects of Climate Change. PLoS ONE, 7(2), e31662. https://doi.org/10.1371/journal.pone.0031662
Influence of climate and reproductive timing on demography of little brown myotis Myotis lucifugus—Frick—2010—Journal of Animal Ecology—Wiley Online Library. (n.d.). Retrieved March 12, 2021, from https://besjournals.onlinelibrary.wiley.com/doi/10.1111/j.1365-2656.2009.01615.x
Jagnow, C. P., Stedman, R. C., Luloff, A. E., Julian, G. J. S., Finley, J. C., & Steele, J. (2006). Why Landowners in Pennsylvania Post Their Property against Hunting. Human Dimensions of Wildlife, 11(1), 15–26. https://doi.org/10.1080/10871200500470944
Jones, R., Travers, C., Rodgers, C., Lazar, B., English, E., Lipton, J., Vogel, J., Strzepek, K., & Martinich, J. (2013). Climate change impacts on freshwater recreational fishing in the United States. Mitigation and Adaptation Strategies for Global Change, 18(6), 731–758. https://doi.org/10.1007/s11027-012-9385-3
Kennedy-Slaney, L., Bowman, J., Walpole, A. A., & Pond, B. A. (2018). Northward bound: The distribution of white-tailed deer in Ontario under a changing climate. Wildlife Research, 45(3), 220–228. https://doi.org/10.1071/WR17106
LCB_Invasive_Species_Guide.pdf. (n.d.). Retrieved April 7, 2021, from http://www.lcbp.org/wp-content/uploads/2012/08/LCB_Invasive_Species_Guide.pdf
Lee, K. V., Steinhauer, N., Rennich, K., Wilson, M. E., Tarpy, D. R., Caron, D. M., Rose, R., Delaplane, K. S., Baylis, K., Lengerich, E. J., Pettis, J., Skinner, J. A., Wilkes, J. T., Sagili, R., vanEngelsdorp, D., & for the Bee Informed Partnership. (2015). A national survey of managed honey bee 2013–2014 annual colony losses in the USA. Apidologie, 46(3), 292–305. https://doi.org/10.1007/s13592-015-0356-z
Liu, Z., He, C., & Wu, J. (2016). The Relationship between Habitat Loss and Fragmentation during Urbanization: An Empirical Evaluation from 16 World Cities. PLOS ONE, 11(4), e0154613. https://doi.org/10.1371/journal.pone.0154613
Loeb, S. C., & Winters, E. A. (2013). Indiana bat summer maternity distribution: Effects of current and future climates. Ecology and Evolution, 3(1), 103–114. https://doi.org/10.1002/ece3.440
Maher, S. P., Kramer, A. M., Pulliam, J. T., Zokan, M. A., Bowden, S. E., Barton, H. D., Magori, K., & Drake, J. M. (2012). Spread of white-nose syndrome on a network regulated by geography and climate. Nature Communications, 3(1), 1306. https://doi.org/10.1038/ncomms2301
Manfredo, M. J., Teel, T. L., Berl, R. E. W., Bruskotter, J. T., & Kitayama, S. (2020). Social value shift in favour of biodiversity conservation in the United States. Nature Sustainability, 1–8. https://doi.org/10.1038/s41893-020-00655-6
McAvoy, T. J., Régnière, J., St-Amant, R., Schneeberger, N. F., & Salom, S. M. (2017). Mortality and Recovery of Hemlock Woolly Adelgid (Adelges tsugae) in Response to Winter Temperatures and Predictions for the Future. Forests, 8(12), 497. https://doi.org/10.3390/f8120497
McClure, C. J. W., Rolek, B. W., McDonald, K., & Hill, G. E. (2012). Climate change and the decline of a once common bird. Ecology and Evolution, 2(2), 370–378. https://doi.org/10.1002/ece3.95
McClure, M. S. (1990). Role of Wind, Birds, Deer, and Humans in the Dispersal of Hemlock Woolly Adelgid (Homoptera: Adelgidae). Environmental Entomology, 19(1), 36–43. https://doi.org/10.1093/ee/19.1.36
Memmott, J., Craze, P. G., Waser, N. M., & Price, M. V. (2007). Global warming and the disruption of plant-pollinator interactions. Ecology Letters, 10(8), 710–717. https://doi.org/10.1111/j.1461-0248.2007.01061.x
Meyer, G. A., Senulis, J. A., & Reinartz, J. A. (2016). Effects of temperature and availability of insect prey on bat emergence from hibernation in spring. Journal of Mammalogy, 97(6), 1623–1633. https://doi.org/10.1093/jmammal/gyw126
Moldowan, P.D., G.J. Tattersall, and N. Rollinson. (2021). Climate-associated decline of body condition in a fossorial salamander. Global Change Biology DOI: 10.1111/gcb.15766.
More total precipitation | Climate Change in Vermont. (n.d.). Retrieved April 12, 2021, from https://climatechange.vermont.gov/our-changing-climate/dashboard/more-annual-precipitation
Morse, J. W., Gladkikh, T. M., Hackenburg, D. M., & Gould, R. K. (2020). COVID-19 and human-nature relationships: Vermonters’ activities in nature and associated nonmaterial values during the pandemic. PLOS ONE, 15(12), e0243697. https://doi.org/10.1371/journal.pone.0243697
Nicholson, C. C., Koh, I., Richardson, L. L., Beauchemin, A., & Ricketts, T. H. (2017a). Farm and landscape factors interact to affect the supply of pollination services. Agriculture, Ecosystems & Environment, 250, 113–122. https://doi.org/10.1016/j.agee.2017.08.030
Nicholson, C. C., & Ricketts, T. H. (2019). Wild pollinators improve production, uniformity, and timing of blueberry crops. Agriculture, Ecosystems & Environment, 272, 29–37. https://doi.org/10.1016/j.agee.2018.10.018
O’Shea, T. J., Cryan, P. M., Hayman, D. T. S., Plowright, R. K., & Streicker, D. G. (2016). Multiple mortality events in bats: A global review. Mammal Review, 46(3), 175–190. https://doi.org/10.1111/mam.12064
Pankhurst, N. W., & Munday, P. L. (2011). Effects of climate change on fish reproduction and early life history stages. Marine and Freshwater Research, 62(9), 1015. https://doi.org/10.1071/MF10269
Pearman-Gillman, S. B., Katz, J. E., Mickey, R. M., Murdoch, J. D., & Donovan, T. M. (2020). Predicting wildlife distribution patterns in New England USA with expert elicitation techniques. Global Ecology and Conservation, 21, e00853. https://doi.org/10.1016/j.gecco.2019.e00853
Peterson, M. N. (2004). An approach for demonstrating the social legitimacy of hunting. Wildlife Society Bulletin, 32(2), 310–321. https://doi.org/10.2193/0091-7648(2004)32[310:AAFDTS]2.0.CO;2
Polgar, C. A., Primack, R. B., Williams, E. H., Stichter, S., & Hitchcock, C. (2013). Climate effects on the flight period of Lycaenid butterflies in Massachusetts. Biological Conservation, 160, 25–31. https://doi.org/10.1016/j.biocon.2012.12.024
Potential effects of climate change on birds of the Northeast | SpringerLink. (n.d.). Retrieved February 22, 2021, from https://link.springer.com/article/10.1007/s11027-007-9126-1
Potratz, E. J., Brown, J. S., Gallo, T., Anchor, C., & Santymire, R. M. (2019). Effects of demography and urbanization on stress and body condition in urban white-tailed deer. Urban Ecosystems, 22(5), 807–816. https://doi.org/10.1007/s11252-019-00856-8
Princé, K., & Zuckerberg, B. (2015). Climate change in our backyards: The reshuffling of North America’s winter bird communities. Global Change Biology, 21(2), 572–585. https://doi.org/10.1111/gcb.12740
RAM, J. L., FONG, P., CROLL, R. P., NICHOLS, S. J., & WALL, D. (1992). The zebra mussel (Dreissena polymorpha), a new pest in North America: Reproductive mechanisms as possible targets of control strategies. Invertebrate Reproduction & Development, 22(1–3), 77–86. https://doi.org/10.1080/07924259.1992.9672259
Regniere, J., Saint-Amant, R., & Duval, P. (2010). Predicting insect distributions under climate change from physiological responses: Spruce budworm as an example. Biological Invasions, 14, 1–16. https://doi.org/10.1007/s10530-010-9918-1
Richardson, L. L., McFarland, K. P., Zahendra, S., & Hardy, S. (2019). Bumble bee (Bombus) distribution and diversity in Vermont, USA: A century of change. Journal of Insect Conservation, 23(1), 45–62. https://doi.org/10.1007/s10841-018-0113-5
Rodenhouse, N. L. R. L., Christenson, L. M. C. M., Parry, D. P., & Green, L. E. G. E. (2009). Climate change effects on native fauna of northeastern forests. Canadian Journal of Forest Research. https://doi.org/10.1139/X08-160
Rustad, L., Campbell, J., Dukes, J. S., Huntington, T., Fallon Lambert, K., Mohan, J., & Rodenhouse, Nicholas. (2012). Changing climate, changing forests: The impacts of climate change on forests of the northeastern United States and eastern Canada (NRS-GTR-99; p. NRS-GTR-99). U.S. Department of Agriculture, Forest Service, Northern Research Station. https://doi.org/10.2737/NRS-GTR-99
Schlesinger, M. D., Corser, J. D., Perkins, K. A., & White, E. L. (n.d.). Vulnerability of At-risk Species to Climate Change in New York. 67.
Schwartz, T. (2014). Cost-Benefit Analysis of Stream-Simulation Culvert. 101.
Slayton, T. (2019, March 20). Hunting helps Vermont’s Forests. Vermont Land Trust. https://vlt.org/forests-wildlife-nature/hunting-helps-forests
Snyder, S. A., Kilgore, M. A., Taff, S. J., & Schertz, J. M. (2008). Estimating a Family Forest Landowner’s Likelihood of Posting against Trespass. Northern Journal of Applied Forestry, 25(4), 180–185. https://doi.org/10.1093/njaf/25.4.180
Townsend, A. K., Sillett, T. S., Lany, N. K., Kaiser, S. A., Rodenhouse, N. L., Webster, M. S., & Holmes, R. T. (2013). Warm springs, early lay dates, and double brooding in a North American migratory songbird, the black-throated blue warbler. PloS One, 8(4), e59467. https://doi.org/10.1371/journal.pone.0059467
Trombly, J. & 2019. (2019, December 29). Vermont tradition in decline: Hunting falls victim to demographic shifts. VTDigger. https://vtdigger.org/2019/12/29/vermont-tradition-in-decline-hunting-falls-victim-to-demographic-shifts/
USGCRP (2018). Impacts, Risks, and Adaptation in the United States: Fourth National Climate Assessment, Volume II: [Reidmiller, D.R., C.W. Avery, D.R. Easterling, K.E. Kunkel, K.L.M. Lewis, T.K. Maycock, and B.C. Stewart (eds.)]. U.S. Global Change Research Program, Washington, DC, USA, 1515 pp. doi: 10.7930/NCA4.2018.
Vayer, V. R., Larson, L. R., Peterson, M. N., Lee, K. J., Furstenberg, R. V., Choi, D. Y., Stevenson, K., Ahlers, A. A., Anhalt-Depies, C., Bethke, T., Bruskotter, J., Chizinski, C. J., Clark, B., Dayer, A. A., Ghasemi, B., Gigliotti, L., Graefe, A., Irwin, K., Keith, S. J., … Woosnam, K. M. (n.d.). Diverse University Students Across the United States Reveal Promising Pathways to Hunter Recruitment and Retention. The Journal of Wildlife Management, n/a(n/a). https://doi.org/10.1002/jwmg.22055
VBBS final report to VFWD 2016. (n.d.). Google Docs. Retrieved July 7, 2021, from https://docs.google.com/document/d/1KOcucBOPyJH7YwWX0uBGCawqBHOKbETLLKlYQN7qL10/edit?usp=sharing&usp=embed_facebook
VT Fish and Wildlife Department. (2020). Big Game Management Plan 2020-2030. Agency of Natural Resources.
VT Fish and Wildlife Department. (2021a). Abenaki Chef Jessee Lawyer to Join Vermont Wild Kitchen March 18 | Agency of Natural Resources. State of Vermont: Agency of Natural Resources. https://anr.vermont.gov/content/abenaki-chef-jessee-lawyer-join-vermont-wild-kitchen-march-18
VT Fish and Wildlife Department. (2021b). Hunting License Sales Dataset 1980-2020. Agency of Natural Resources. Accessed July 27th, 2021.
Walberg, E., Cornicelli, L., & Fulton, D. C. (2018). Factors impacting hunter access to private lands in southeast Minnesota. Human Dimensions of Wildlife, 23(2), 101–114. https://doi.org/10.1080/10871209.2018.1396510
Water Q&A: What causes fish kills? (n.d.). Retrieved July 29, 2021, from https://www.usgs.gov/special-topic/water-science-school/science/water-qa-what-causes-fish-kills?qt-science_center_objects=0#qt-science_center_objects
Weiskopf, S. R., Ledee, O. E., & Thompson, L. M. (2019). Climate change effects on deer and moose in the Midwest. The Journal of Wildlife Management, 83(4), 769–781. https://doi.org/10.1002/jwmg.21649
Wells, M. L., Trainer, V. L., Smayda, T. J., Karlson, B. S. O., Trick, C. G., Kudela, R. M., Ishikawa, A., Bernard, S., Wulff, A., Anderson, D. M., & Cochlan, W. P. (2015). Harmful algal blooms and climate change: Learning from the past and present to forecast the future. Harmful Algae, 49, 68–93. https://doi.org/10.1016/j.hal.2015.07.009
Wetlands as Bird Habitat. (n.d.). Retrieved July 7, 2021, from https://water.usgs.gov/nwsum/WSP2425/birdhabitat.html
Wilderness brook trout | Vermont Fish & Wildlife Department. (n.d.). Retrieved July 29, 2021, from https://vtfishandwildlife.com/wilderness-brook-trout
Wilson, E. C., Shipley, A. A., Zuckerberg, B., Peery, M. Z., & Pauli, J. N. (2019). An experimental translocation identifies habitat features that buffer camouflage mismatch in snowshoe hares. Conservation Letters, 12(2), e12614. https://doi.org/10.1111/conl.12614
Winkler, R., & Warnke, K. (2013). The future of hunting: An age-period-cohort analysis of deer hunter decline. Population and Environment, 34(4), 460–480. https://doi.org/10.1007/s11111-012-0172-6
Wiseman, F. M. (2001). The Voice of the Dawn: An Autohistory of the Abenaki Nation. UPNE.
Xu, C., Letcher, B. H., & Nislow, K. H. (2010). Context-specific influence of water temperature on brook trout growth rates in the field. Freshwater Biology, 55(11), 2253–2264. https://doi.org/10.1111/j.1365-2427.2010.02430.x
Zebra Mussels | Vermont Fish & Wildlife Department. (n.d.). Retrieved April 7, 2021, from https://vtfishandwildlife.com/learn-more/landowner-resources/liep-invasive-species-program/aquatic-invasive-animals/zebra-mussels
Zebra Mussels | Vermont Invasives. (n.d.). Retrieved April 7, 2021, from https://vtinvasives.org/invasive/zebra-mussels
Zuckerberg, B., Bonter, D. N., Hochachka, W. M., Koenig, W. D., DeGaetano, A. T., & Dickinson, J. L. (2011). Climatic constraints on wintering bird distributions are modified by urbanization and weather. Journal of Animal Ecology, 80(2), 403–413. https://doi.org/10.1111/j.1365-2656.2010.01780.x