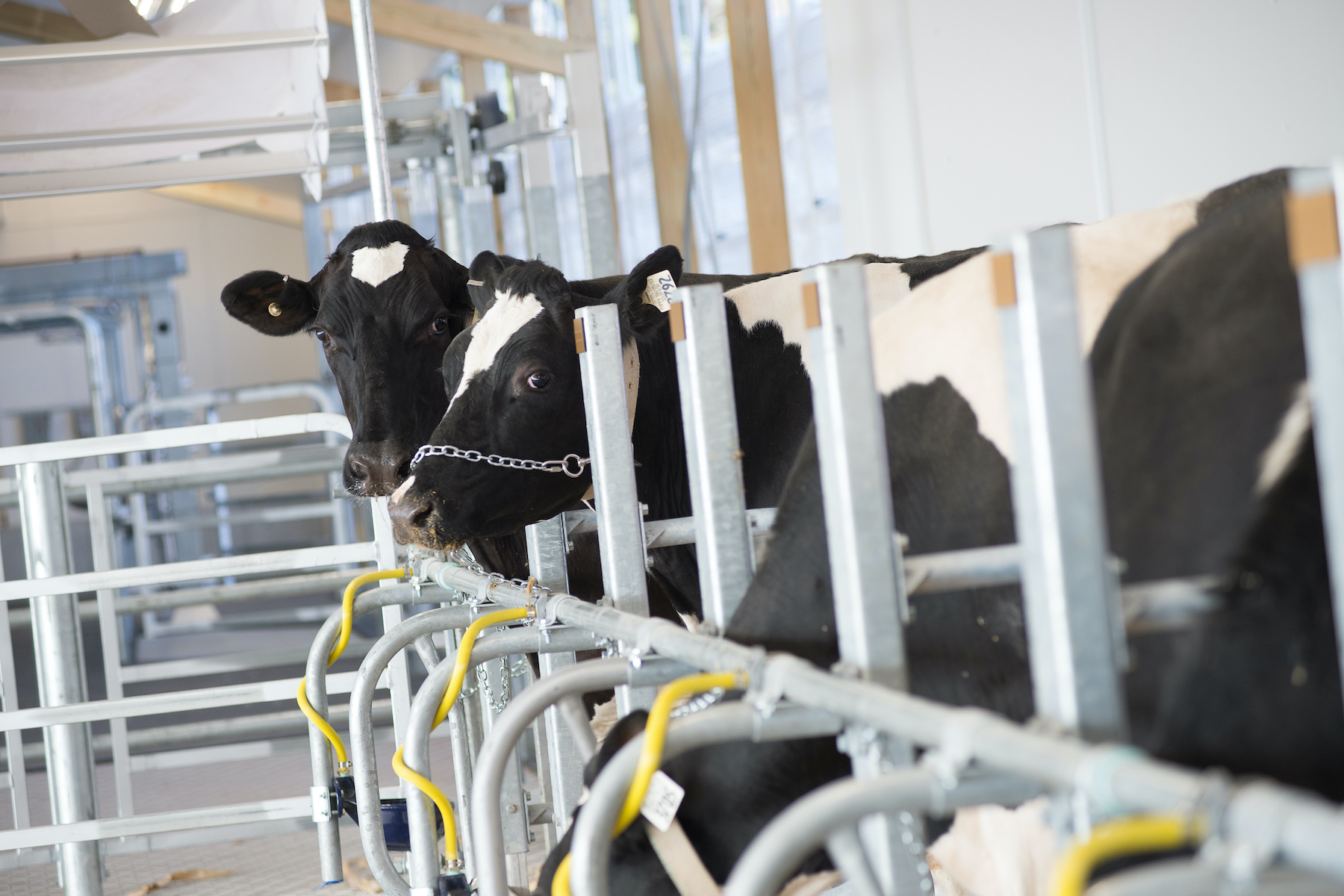
Agriculture and Food Systems
Download chapter PDF and figures
Authors
Ryan Ruggiero
Department of Plant and Soil Science, University of Vermont, Burlington, VT 05405
Joshua Faulkner
Department of Plant and Soil Science, University of Vermont, Burlington, VT 05405
Department of Civil and Environmental Engineering, University of Vermont, Burlington, VT 05405
Farming and Climate Change Program, University of Vermont Extension, Burlington, VT 05405
Gund Institute for Environment, University of Vermont, Burlington, VT 05045†
Alissa White
Department of Plant and Soil Science, University of Vermont, Burlington, VT 05045
Jake Claro
Farm to Plate, Vermont Sustainable Jobs Fund, Montpelier, VT 05602
Carina Isbell
Department of Community Development and Applied Economics, University of Vermont, Burlington, VT 05405
Citation
Ruggiero, R., Faulkner, J., White, A., Claro, J., Isbell, C. 2021. Agriculture and Food Systems. In Galford, G.L., Faulkner, J. et al. (Eds), The Vermont Climate Assessment 2021. Burlington, Vermont: Gund Institute for Environment at the University of Vermont. DOI: 10.18125/kowgvg.
Table of Contents
- 5.1 Key Messages
- 5.2 Overview
- 5.3 Agriculture and Food Systems in a Changing Climate
- 5.4 Mitigation, Resilience, and Adaptation
- 5.5 Traceable Accounts
- 5.6 References
5.1 Key Messages
- Vermont’s climate is already changing in ways that benefit its agricultural system, including longer growing periods (freeze-free periods lengthened twenty-one days since early 1900s) and milder temperatures (annual average temperature increase of 2°F (1.1°C) since the 1990s), allowing farmers to experiment with new crops or practices not previously viable in Vermont.
- The changing climate also brings agricultural setbacks, such as negative impacts on fruit-bearing species like apple trees that require a sufficient over-wintering period for success in the next growing season. The maple syrup industry is also at risk due to variations in winter temperatures.
- Climate models predict tougher growing conditions due to greater variability in temperature and precipitation, including heavy precipitation and dry spells.
- Vermont’s average annual precipitation has increased 6.7 inches since the 1960s. Summer precipitation has increased most (additional 2.6 inches since 1960s) and is characterized by more heavy precipitation events (defined as more than one inch of precipitation in one day), although spring precipitation has also increased notably (additional 2.11 inches/year since 1960s, and 0.8 days/year with heavy precipitation). Spring precipitation accumulates in the soil and can make farm operations difficult. While precipitation during the growing season is trending upward, precipitation falls in fewer, more extreme events and is coupled with longer periods of no rain at times when crop water requirements are still high; thus, irrigation may become increasingly important.
- At the Earth’s surface, increasing concentrations of carbon dioxide may benefit yields in crops that utilize the C3 photosynthetic pathway (i.e., many of Vermont’s forages) if conditions are otherwise ideal. Conversely, an increase in surface-level ozone concentrations may reduce crop productivity.
- Extreme events are expected to increase. More periods of flooding and drought will lead to more crop damage or failure. Stormwater and irrigation infrastructure will be crucial in mitigating these effects.
- Agriculture and food systems may play an important role in mitigating climate change, if mitigation provides financial opportunities, are distributed fairly and accurately, and are implemented with careful monitoring, reporting, and verification. Urban and suburban areas in Vermont have the potential to improve adaptation and mitigation of climate change by growing food closer to where it is consumed.
5.2 Overview
Agriculture has been, and remains, a defining characteristic of Vermont’s landscape, culture, and economy. The state’s food system has experienced tremendous growth over the past decade, as evidenced by the expansion of the food system’s economic output by 48% from 2011 to 2020 (Kavet, 2020). By 2017, 13.9% of all in-state food purchases were comprised of Vermont’s farm and food products (Willard et al., 2020). Despite this growth, climate change has the potential to significantly disrupt farm and food system profitability and viability. Multiple climate shocks are possible within single growing seasons, and climate change impacts occurring elsewhere in the country can have ripple effects in Vermont. Resilience measures are critical across this sector, but they will vary based on the specific type of enterprise or production system. Farmers and food system workers are not unfamiliar with challenges and change, and they have already demonstrated instances of successful and ingenious adaptation. As the data in this chapter show, individuals and businesses must operate and move forward knowing that they cannot plan for what was once known as “normal.”
5.3 Agriculture and Food Systems in a Changing Climate
Impending climate change will increase precipitation and temperature variability and extreme events. These changes have the potential to both positively and negatively affect production agriculture and food systems in Vermont. Potential benefits and drawbacks are discussed in the following sections.
5.3.1 Freeze-Free Period
The freeze-free period is defined as the number of consecutive days in which the minimum temperature does not go below 28°F (-2.2°C). The length of the freeze-free period is not the same as the length of the growing season. The freeze-free period is used to assess how climate change will affect production agriculture. Statewide data from climate stations (see Climate Change in Vermont chapter) show a significant increasing trend in the length of the freeze-free period across the state. From 1960 to 2020, the freeze-free period increased at an average rate of 4.4 days per decade and accelerated to 9.0 days per decade since 1990 (Figure 5-1). Since the 1990s, the most dramatic freeze-free increase is observed in the Southern and East/Northeastern portions of the state (increase of 17 days and 18 days, respectively); the Western portion has increased 10 days. Farmers in Western Vermont (i.e., Champlain Valley) have experienced relatively modest changes, whereas farmers east of the Green Mountains and to the south are dealing with conditions that are quite different from what was “normal” thirty to fifty years ago. The historical observations suggest a strong trend that may continue over the next several decades. With continued climate change, the entire state can expect longer average freeze-free periods. However, farms— depending on their location—will have more pronounced impacts
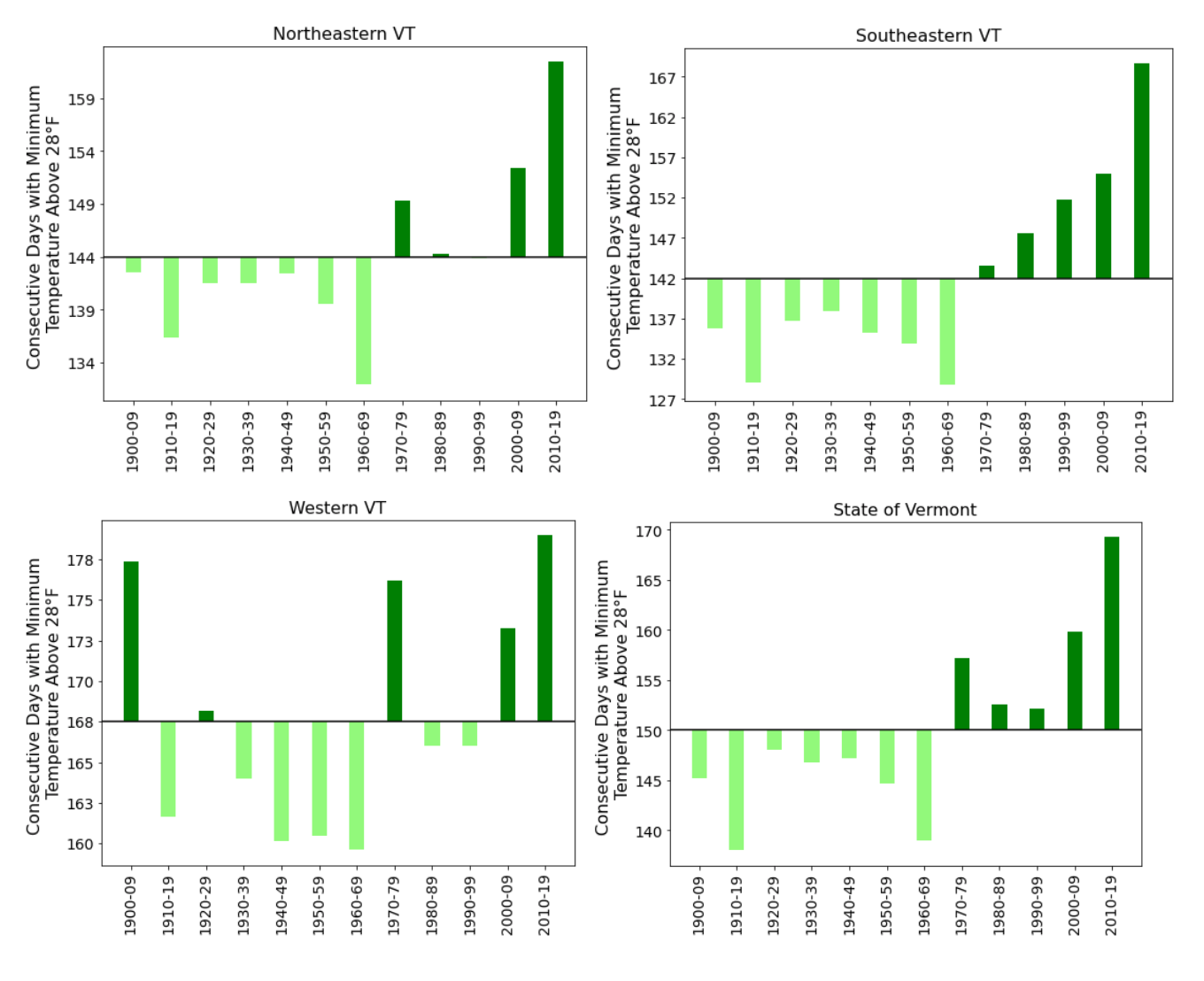
Note: The freeze-free period is calculated based on the number of consecutive days with minimum temperature above 28ºF (-2.2°C). In this figure, the decadal average is plotted above or below the 1900–2019 mean (solid black line) for a) Northeastern Vermont, b) Southeastern Vermont, c) Western Vermont, and d) State of Vermont (Figure 1-10, Climate Change in Vermont chapter). Note the differences in y-axis ranges.
Simulations of future freeze-free periods from a conservative climate change scenario (RCP 4.5) estimate an additional 12-20 days by 2069 relative to 1979-2008 (Figure 5-2). This may lead to increased yields for farmers and/or allow for cultivation of new crops that previously would not have been successful in Vermont. The list of new crop options is likely to evolve over time, as farmers and researchers explore the possibilities. For example, efforts to grow saffron outdoors are underway, and research shows it is economically viable in the state (https://www.uvm.edu/~saffron/). Saffron is the most expensive spice in the world and previously was grown only in warm climates near the Mediterranean and in India.
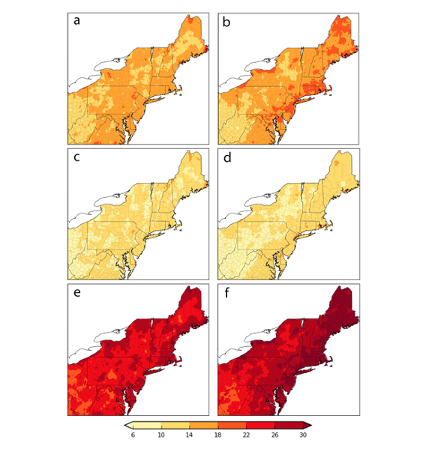
Note: Freezing is defined as -32°F (0°C). Comparison period is 1979-2008. Models used are: a, b) 2040–2069 RCP8.5; c, d) 2040–2069 RCP4.5; and e, f) 2070–2099 RCP8.5. Adapted from Wolfe et al. 2018.
A later first-frost will extend the growing season for both annual and perennial crops in Vermont (Wolfe et al., 2018). This will increase harvest flexibility, giving forage crops extra time to accumulate biomass, especially important if planting was delayed in spring. Late first fall frosts may create opportunities for later harvests (i.e., tomatoes, cut flowers, fall raspberries, or additional cuttings of hayfields). It may also be possible for double cropping (two crops per field in one growing season) to occur. Other factors, such as excess moisture, also affect spring farming practices. Bare soils prior to planting in the spring do not have plants to return soil moisture to the atmosphere through transpiration, so moisture accumulates in the soil and increases risks of compacting soil or damaging (i.e., rutting) fields if heavy machinery is operated (i.e., to terminate a cover crop or plant) or of damaging machinery. Thus, a longer freeze-free period does not necessarily mean farmers will be able to plant sooner. The benefit of a longer growing season may be offset by the trends in springtime precipitation.
5.3.2 Water
5.3.2.1 Spring Precipitation
Previous trends in spring precipitation in New England show that bulk precipitation in the twenty-one days leading up to the spring thaw has increased (Figure 5-3). Climate models project this trend will continue at an alarming rate (Wolfe et al., 2018). This is a classic representation of how climate change is impacting Vermont and the rest of the world: the extremes become more extreme. Spring is notorious in Vermont for being the wet (mud) season, and that is likely to stay the case as the climate changes.
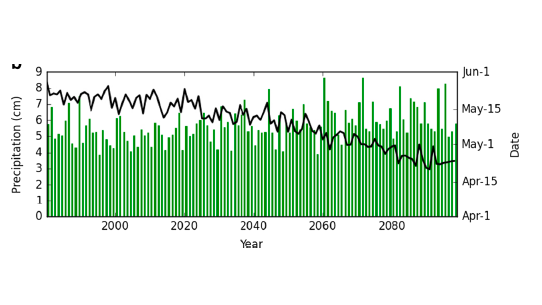
Note: Data is from observed historical data and simulated future climate scenario based on RCP8.5. Adapted from “Unique challenges and opportunities for northeastern US crop production in a changing climate” by Wolfe et al., 2018).
5.3.2.2 Tile Drainage
Farmers in Vermont have adapted to the wet conditions in spring by using artificial subsurface tile drainage. Originally used to drain wetlands in the midwestern United States, tile drains are perforated plastic pipes that are installed to a depth of one meter to remove excess water from the soil, thereby improving farm operations and crop productivity (Madramootoo, 1999). Vermont farmers who have not tiled their fields may do so to adapt to climate change. Usually tile drains are used on finely textured, poorly drained soils that are at risk for low yield and soil compaction because of excess moisture. Many of the farms in the Champlain Valley are located on these types of soils, and tile drainage has been shown to be an effective method for improving trafficability and growing conditions in field crops and vegetable systems (King et al., 2015). Tile drainage systems also have been shown to transport nutrients more rapidly through the subsurface, raising water quality concerns. To address these concerns, alternative soil and nutrient management practices can be implemented, or tile drainage systems can be modified with drainage water management structures to control the height of the water table in fields. Some studies have found that these structures reduce nutrient and sediment loss during extreme events (Tan and Zhang, 2011). Other methods of removing excess water include surface-grading and ditching fields. Research regarding the environmental impacts of tile drainage systems is ongoing in Vermont (Moore, 2016).
5.3.2.3 Water Stress and Irrigation
As discussed in the Climate Change in Vermont chapter of this report, summer precipitation has increased in recent decades, but it has also become increasingly variable. Timing of precipitation during summer months is and will continue to be irregular. It is likely that heavy precipitation events (greater than one inch of precipitation per day) will supply much of summer precipitation, which means farmers will need to adjust their stormwater infrastructure for larger runoff events. Farmers can work with state and federal agencies to engineer, permit, and cost-share stormwater infrastructure improvements.
Farmers also need to prepare for increased frequency of prolonged dry spells or droughts. Even if farmers invest more in irrigation infrastructure, there might be limitations on water access. While summer stream low flow has not shown any significant decreasing trend in Vermont (see Water Resources chapter), reduced pond storage and dry wells may occur in years of drought, and stream base flow may be reduced. It is worth noting that most wells in Vermont are not on highly desirable geology; that is, they are not apt to be high producing water sources for future water needs (see Water Resources chapter). Farmers cannot make surface withdrawals from streams when flow rates fall below the threshold set for preserving wildlife (Armstrong et al., 2001). While it is difficult to anticipate the timing of individual farms’ water stress, periods of crop water stress are likely to occur for farms simultaneously throughout a region. Farmers can prepare for low water availability by using seasonal potential evapotranspiration (PET) computer simulation models, staying current with the Vermont drought conditions monitor (https://www.drought.gov/states/vermont/), and working with other farms to strategically plan water delivery services. Farmers should also consider using remotely monitored soil moisture sensors to understand how stressed their crops are in real time. Cost share programs would be beneficial to allow farmers to employ this equipment without as much financial and operational burden.
Water for irrigation may remain sufficient for Vermont producers; however, the temporal distribution and disruptions of future precipitation are subject to much variation. Recent trends show increasing precipitation in all seasons, with the greatest rate of increase in summer precipitation, and they show increased variability and frequency of dry spells (see Climate Change in Vermont chapter). Increasing precipitation does not mean farmers will require less irrigation; in fact, sprinkler irrigation in Vermont has increased from 1985 to 2015 at the same time precipitation increased (Figure 5-4) (USGS, 2021b; see Climate Change in Vermont Chapter). It is likely the need for irrigation will continue to increase because of increasingly variable precipitation. Heavy precipitation events are expected to bring much of any given season’s total precipitation, which is not as helpful during the growing season as frequent, smaller precipitation events. The length of dry periods will also increase, which may force farmers to irrigate (Trenberth, 2011). Climate models suggest that by 2050 the amount of monthly precipitation during the growing season (summer) will be less on average than in the period 1980-1999 (Figure 5-5) (USGS, 2021a)
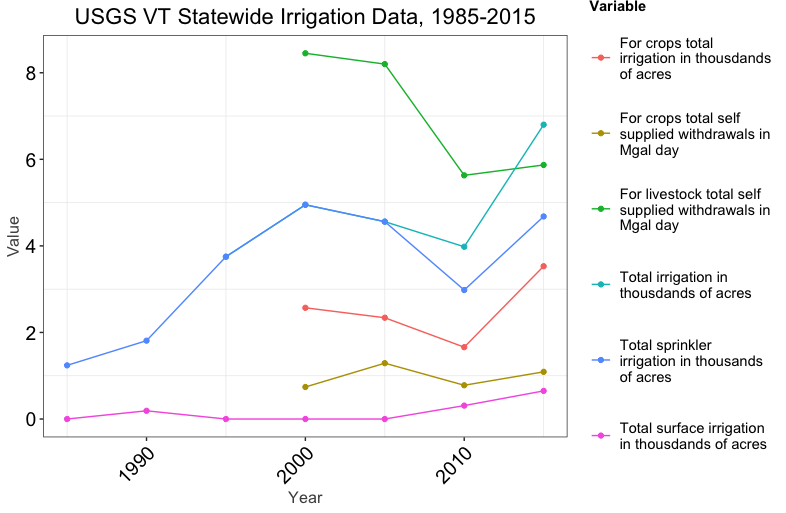
Note: Data represents surface and groundwater combined withdrawals (self-supplied) reported every 5 years (USGS, 2021b).
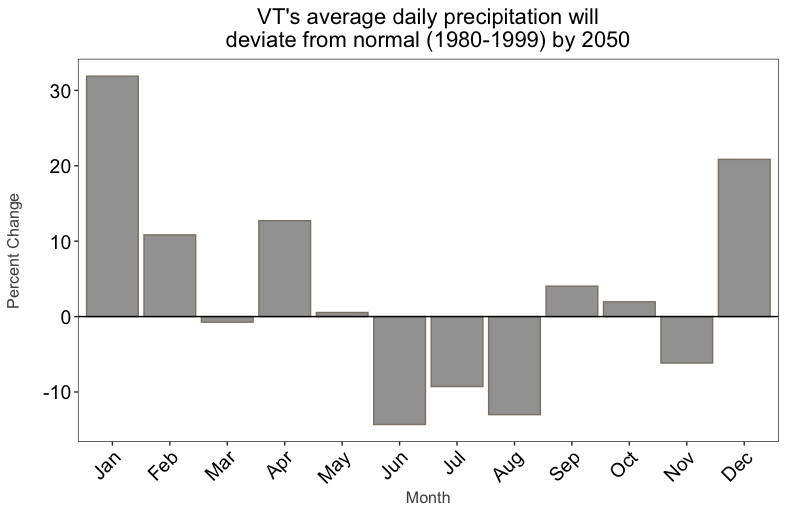
Groundwater and surface water withdrawals for livestock decreased from 8.45 million gallons (Mgal) per day in 2000 to 5.87 Mgal per day in 2015, though an increase was observed from 2010 to 2015 (Figure 5-4) (USGS, 2021b). In Vermont, self-supplied water withdrawals for livestock are greater than that for crops. Peak groundwater usage may increase during summer months when surface water withdrawal from streams and ponds are no longer available due to limits for wildlife conservation. While trends in overall moisture suggest there will be sufficient groundwater in Vermont, farms will still need to adapt to the increased energy costs associated with higher rates of groundwater extraction.
5.3.2.4 Flooding
Increased precipitation, particularly periods of heavy precipitation events, are leading to increased flooding in Vermont (see Water Resources chapter). Farms located within floodplains will continue to be at risk as more extreme and unpredictable flood events occur. In addition, farms outside of floodplains (designated 25- or 50-year return period) may experience more frequent floods (Wolfe et al., 2018). Flooding during the growing season can be devastating— due to potential contamination with human waste, heavy metals, etc., even crops that survive may not be allowed to enter the human or animal food supply if the edible portion has been in contact with flood waters. If flood waters have not directly contacted the edible portion, there are certain measures farmers can take to ‘save’ the crop; however, usage is determined on a case-by-case basis. Flooded produce is covered in depth by the UVM Extension FAQ about handling flooded produce (Nickerson et al., 2013).
Box 5.1: Halloween 2019 Floods in Vermont
A 2019 Halloween storm created major agriculture losses, even though the event occurred outside of the major growing season. For example, Maple Wind Farm in Richmond lost 2,000 turkeys and chickens (unfortunate timing due to the approaching Thanksgiving holiday) when the Winooski River rose at an astonishing rate. This contributed to a significant loss of income during the winter season. Other farms, like Boston Post Dairy Farm, had haybales displaced and contaminated by floodwaters.
However, impacts were minimal at the Intervale Farm, where farmers gathered last minute support from the Burlington community to mass harvest remaining frost-hardy crops before the flood hit. As this area is within the known Winooski floodplain, farmers there are accustomed to watching the river hydrograph for rising floodwaters. All farms in floodplains could benefit from flood mitigation protocols like those used at the Intervale.
5.3.2.5 Water Stress
Drought conditions will become more variable, with water deficits occurring and accumulating during the non-growing season when soils and surface waters are typically recharged. Droughts often carry over from one year to the next, or a drought may begin in the winter, such as in parts of Vermont in 2002, 2012, 2013, 2015, and 2017. As of May 2021, 75% of the state was in moderate drought, and the entire state was classified as abnormally dry (National Integrated Drought Information System, n.d.). Droughts also occurred in much of the state during the 2016, 2018, and 2020 growing seasons. In 2020, farmers in at least ten Vermont counties were eligible for federal aid for crop loss due to the drought; losses were estimated at $27 million (McCallum, 2020).
5.3.3 Warmer Temperatures and Temperature Variation
During the growing season, warmer temperatures, including heat waves and warmer overnight temperatures, are expected (see Climate Change in Vermont chapter). Warmer temperatures benefit existing crops, offer success with new crops, and may make double cropping possible. Dairy farmers may be able to plant more productive field crop varieties as well. Warmer temperatures will also result in increased livestock stress and a need for additional cooling infrastructure, including shade structures for pasture-based operations. Warmer temperatures also promote evapotranspiration and can stress crops at vulnerable times.
Crop stress is likely to increase during the spring because of increased variation in springtime temperatures and will have the greatest impact on cash crops. Once fruiting crops meet their winter chilling requirements, they will bud once temperatures warm, but cold temperatures following a warm period can damage buds or even cause crop failures (Stafne, 2020). Warm winter days, defined as days with temperatures of greater than 50°F (10°C), in Vermont have increased by 1.82 days between the 1960s and 2020. Cold winter nights, defined as a minimum temperature of 0°F (-17.8°C), have decreased more substantially, by10.01 days (see Climate Change in Vermont chapter). Trends suggest warmer winters will continue in Vermont, although it may be several decades before crops like apples (which has one of the strongest chilling requirements of fruiting bearing species) will suffer the effects of insufficient overwintering. This may be a problem first in southern Vermont, where warming trends are more pronounced. The most pressing issue all farmers will have to deal with is fluctuations in late winter/early spring temperatures that result in budding with subsequent frost periods. This can be devastating for crops that respond more rapidly to brief periods of warming, so farmers will have to adapt by selecting varieties that tolerate temperature fluctuations better (Wolfe et al., 2018). Also see the following section on pest pressures
5.3.4 Changing atmospheric conditions and pest pressures
Globally, pest pressures on agriculture are changing with climate, providing clues to the challenges Vermont may face. Despite improvements to pest and disease management technology, global crop production loss due to pathogens is estimated at 10-16% (Chakraborty and Newton, 2011). A challenge caused by climate change is shifting atmospheric conditions, specifically concentrations of CO2 and ozone that can affect crop diseases and productivity. Pests and diseases with expanded range, increased competitiveness, or other advantages are expected to put increasing pressure on crops as well. A lack in genetic diversity of cash crops means they will fatigue quicker than weeds, pests, and diseases, which can mutate and adapt more rapidly to climate change (Raza et al., 2019).
Continued increases in atmospheric CO2 concentrations can enhance photosynthesis and may result in higher yields for crops (i.e., high quality forages). The benefits from increased CO2 may be offset by the damage occurring from increased surface-level ozone, which can increase disease expression and thus decrease crop productivity (Eastburn et al., 2010). Ozone-related damage and decreased productivity varies with crop species—many Vermont crops are considered ozone-sensitive, including forages, fruit bushes, grapes, lettuce, potato, spinach, tomato, and watermelon. In contrast, certain vegetable crops like strawberries and tomatoes grown in the presence of elevated ozone had increased concentrations of nutrients like vitamin C and β-carotene, respectively (Moretti et al., 2010).
The impact climate change will have on pests is a function of the expected increase in CO2 concentrations and temperatures. One plant response to increased CO2 is an increase in foliar carbon:nitrogen ratios. For example, reduced foliar nitrogen in bell pepper plants due to elevated CO2 concentrations caused reproduction setbacks for phloem-feeding insects by 37%, yet their feeding was unaffected (Dáder et al., 2016). While some leaf-chewing insects have been found to suffer reproduction setbacks up to 34%, other insects in this category may benefit (Robinson et al., 2012). For hemipterian species (also known as “true bugs”) that feed on plant sap, increased CO2 resulted in both increased and decreased animal counts, depending on the hay and garden crops and the different species of this order (Guo et al., 2014; Oehme et al., 2013). These studies indicate that some pests will have reproductive setbacks, but others may benefit.
Temperature changes may impact insects’ life cycles and activities. Some insect orders cannot reproduce beyond a certain temperature threshold, so increased temperatures will result in reduced populations. However, insects may be able to adapt their reproductive window or increase their temperature thresholds if change occurs gradually (Debarro and Maelzer, 1993). Insects also have an upper temperature threshold for flight, which will ultimately affect migration and daily activity (Trębicki and Finlay, 2018). Temperature changes also can influence the number of generations and species distribution (Trębicki and Finlay, 2018).
Insects in Vermont are likely to be impacted in the same physiological ways; however, the degree of pest adaptation is still unknown. Vermont can expect an increase in new pest and disease species that formerly were limited to southern latitudes; this already has been the case with more mild non-growing season conditions in the forest sector (see Climate Change in Forests chapter). More research and a holistic approach to pest and disease management are required for Vermont to best adapt to climate change.
The uncertainty around pest changes is similar for disease changes. For example, the threat of fire blight caused by the bacteria (Erwinia amylovora) increases with warmer spring temperatures, resulting in greater risk of earlier blooming during rain events for crops such as apples and pears. After entering the plant via the flower, fire blight kills vascular plant tissue, leading to a loss of limbs or whole tree mortality. In Switzerland, Hirschi et al. (2012) showed that the effect of earlier blooming on fire blight was offset by changing trends in spring precipitation in some regions of the country, while the southern regions had significant increases in fire blight. As a sporadic and climate-related disease, the impact of fire blight may be erratic in Vermont, but it is known to occur increasingly in New England (Bradshaw, 2016).
5.4 Mitigation, Resilience, and Adaptation
This section discusses how the agricultural and food systems in Vermont can adapt to climate change, informed by how other systems around the world have and continue to adapt.
5.4.1 Food Systems in a Climate-Conscious State
Vermont’s food system is critical to its economy, identity, and quality of life. While food is rightfully associated with farming, the food system in its entirety encompasses a complex network of resources, activities, and people that extend beyond our farmland. The food system, in addition to farmers and farmland, includes processing; distribution; various market channels and their consumers (e.g., farmer’s markets, institutions, grocery stores, restaurants); food waste management; the support system of nonprofits, government agencies, educational institutions, and funders and investors; and the people (e.g., farmers, food workers, policy makers, consumers) involved in all these activities. From an economic perspective, these interacting pieces generate $11.3 billion and employ more than 64,000 Vermonters. In one way or another, we are all participants in the Vermont food system.
Given the significant footprint of the food system in Vermont; its importance in contributing to the state’s and region’s food security; and its far reaching influence on our economy, environment, and culture; Vermont’s ability to successfully mitigate and adapt to climate change requires an integrated understanding of the myriad ways in which the food system contributes to, is affected by, and can provide mitigative, adaptive, and resilient climate solutions for Vermont in what is an era of increasing climate disruption. Developing a climate-smart food system cannot solely rely on policies, innovation, and management focused on the farm gate—though farm-based climate solutions are necessary—but it also must account for and address interdependencies among inputs, production, processing, distribution, point of sale (i.e., markets), consumption, and waste management. For example, in examining where food is coming from and what is coming from elsewhere, the state can begin to imagine and plan for scenarios in which food access decreases or is at risk due to food chain disruptions in other parts of the country (due to crop failures, labor shortages, damaged infrastructure etc.). The food flows analysis provides a window into the complexity of America’s food system and Vermont’s (and New England’s) reliance on certain regions or states (Figure 5-6).
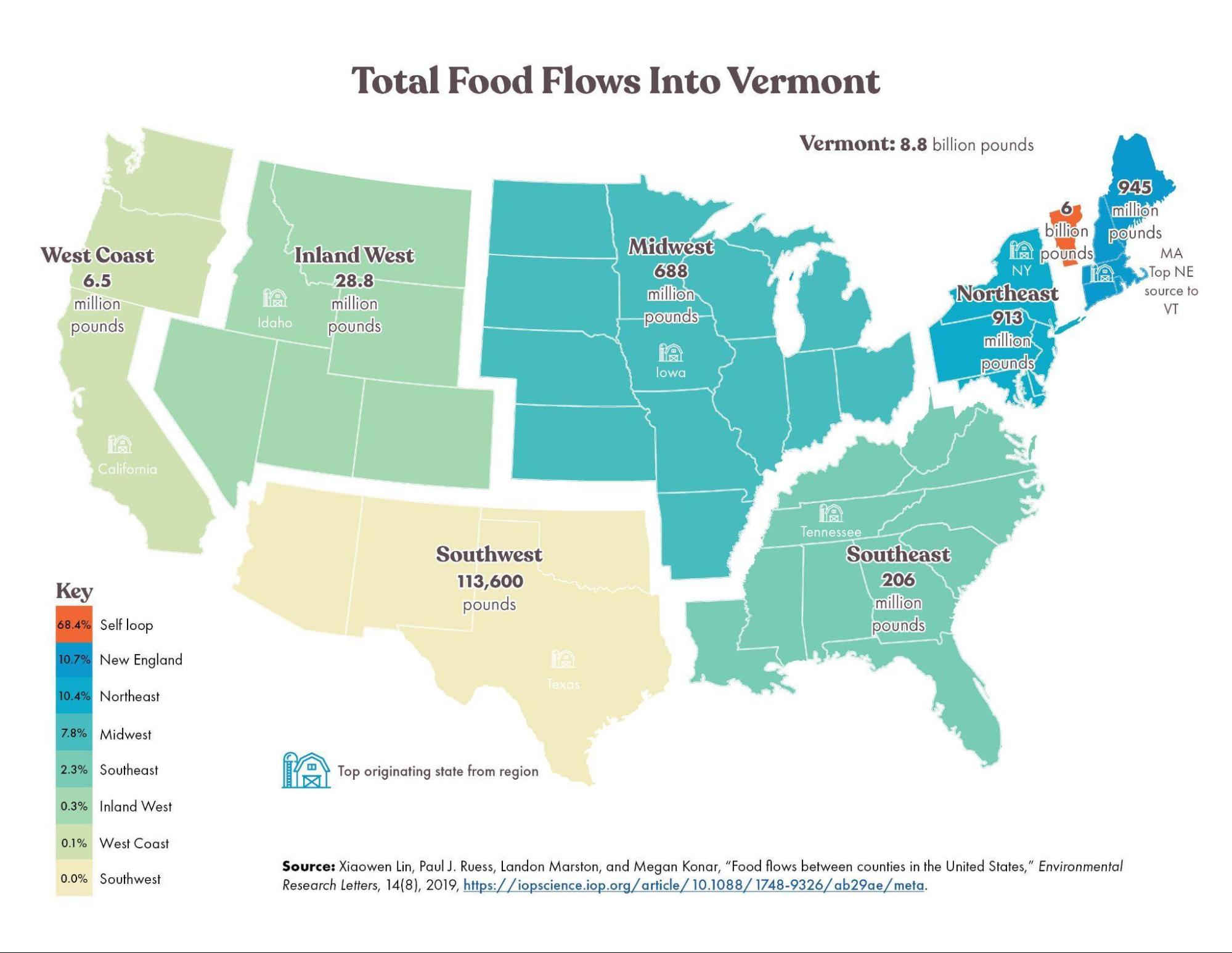
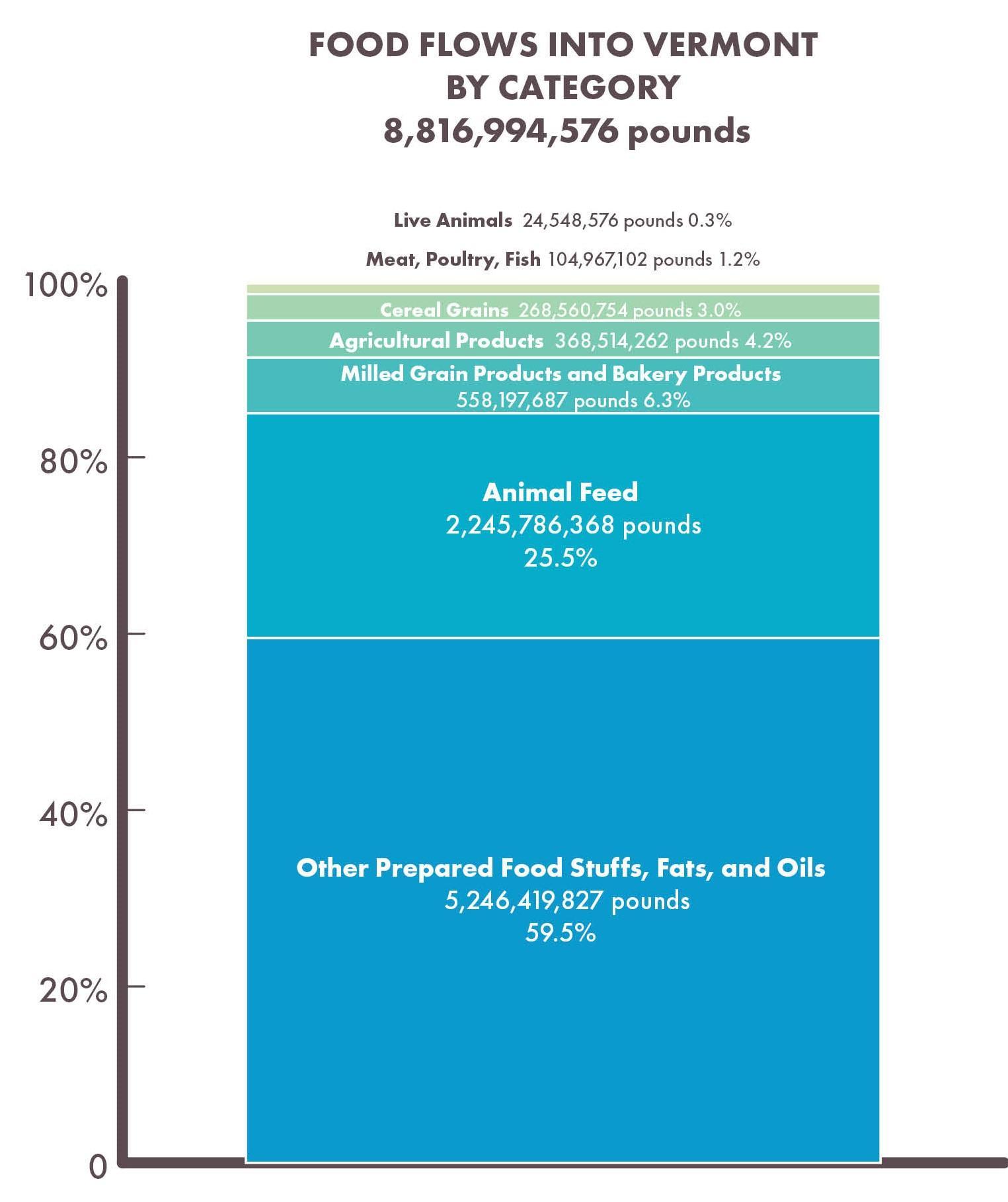
Note: The food flows analysis is about the transportation of food, not about production or consumption per se. It’s suggestive of an order of magnitude of food that enters and moves around between and within states, helping to better conceptualize supply chain vulnerabilities and risks of regions, particularly as they relate to climate change, economic disruption, and Covid-19 or future pandemics. Modified after Lin et al. 2019.
Each component of the food systems supply chain has a part to play in mitigating climate change, but each component also must be prepared to adapt to and withstand the impacts of climate to ensure the viability and security of the food supply. For example, food manufacturing—Vermont’s second largest manufacturing industry—can improve mitigative capacity by adopting more energy-efficient processes and technologies and utilizing renewable energy. Planning and investment are also needed to increase the industry’s adaptive capacity through: making facilities more resilient to extreme weather (particularly flooding), improved packaging that reduces waste and maintains quality and safety (particularly in extreme heat), and diversifying ingredient suppliers through local and regional supply chain development to reduce over-reliance on global supply chains that will face climate disruption. This is one example; of course, there are many more examples to draw from and questions that arise as the entire food supply chain is examined. For example, how can transportation emissions be reduced through accelerated adoption of electric vehicles and more efficient aggregation and storage infrastructure? How can distribution be more adaptive and resilient to climate disruption through greater logistics coordination? How can roads themselves be improved to withstand extreme weather to limit delivery delays to both commercial and charitable food sites? In what ways can energy use, particularly for refrigeration, be more efficient? What kind of additional incentives, technical and business support services, and training are needed to help farm and food businesses address these issues?
These are all critical questions to answer, yet beyond farming there is a relative dearth of available data regarding baseline food system emissions and potential for emission reductions and little evaluation of the preparedness and adaptive capacity of food processing, distribution, point of sale, and waste management systems. However, Vermont’s Agriculture and Food System Strategic Plan 2021-2030 provides a roadmap to address these questions in a systemic way by articulating the vision, goals, and strategies that aim to put the state on a path to developing a climate-smart food system by 2030 (Willard et al., 2020). To do so, priority strategies from the Plan emphasize the need for increased, improved, and energy-efficient processing infrastructure; greater aggregation and distribution coordination and infrastructure; increased incentives and research for climate-adaptive practices; food security planning that ensures the Vermont food supply is sufficient to withstand global or national food supply chain disruptions caused by climate change; food system mapping that accounts for predicted climate impacts in order to aid state and municipal planning and investments; and increased professional development for technical assistance and business planning providers in order to help their farm and food business clients address and integrate climate change issues into management decisions and long-term planning.
5.4.2 Adaptation
Though farmers in Vermont have some options when it comes to adapting to climate change, economics emerges as a major barrier to adaptation, as it currently is cheaper for farmers to operate in a business-as-usual manner. Many farmers are climate conscious because they understand the impact climate has on their bottom line (Figure 5-7) (USDA FSA). Cost sharing, or government payments to help install conservation practices, is a way farmers can experiment with well researched best management practices (BMP) that will increase resilience to and mitigation of climate change with less of a financial burden. Farmers would rather preserve the environment than put it in jeopardy, but they only can adopt practices if they are able to financially.
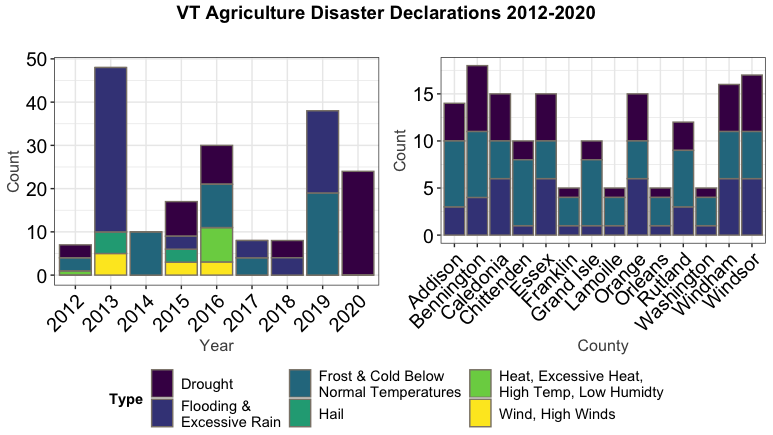
Note: Some counties have more than one disaster type per year. Source: USDA FSA.
5.4.3 Vermont Farmer Survey Data
Vermont farmers are adapting to climate change impacts based on previous experiences with weather extremes and perceptions of increases of extreme weather. Of the sixty-four Vermont farmers who responded to the New England Adaptation Survey, 65% made management changes due to an experience with drought, 56% were planning to make changes to their farms to manage for the risk of increased incidence of drought, 77% made changes due to experiences with extreme precipitation and flooding, and 64% were planning to make changes to address the risk of increased incidence of extreme precipitation events.
Farmers have primarily invested in soil health and conservation practices to help them address the risks of extreme precipitation associated with climate change (Figure 5-8). Additionally, farmers identified a wide range of practices and strategies they planned to employ based on their experiences with extreme weather, from changes in cropping systems to water catchment systems, irrigation, and hoop houses (White et al. 2018). While crop insurance is a strategy employed by large farmers in other regions (Mase et al. 2017), small and diversified farms in Vermont report low rates of crop insurance use to address weather extremes (Figure 5-8).
Most farmers in the region understand their vulnerability to the extreme weather associated with climate change, but they report that they lack the technical skills and financial capacity to adequately address climate-related risks and invest in adaptation (White et al. 2018). Research in Vermont identifies a gap in access to consistent and committed financial tools to support farmers’ capacity to adapt to climate change (White, 2021; White et al., 2018). In focus groups, producers identified alternative financial safety nets such as community-supported agriculture groups and emergency relief funds as critical to supporting financial viability of their small and diversified farms (White and Pankoff, 2019). Farmers have also identified interest and willingness to participate in payment for ecosystem services (PES) schemes to address both climate adaptation and mitigation (White, 2021; White and Faulkner, 2019). In the last few years, a wide range of stakeholders has put effort into exploring the real potential for a healthy soils PES system to meet multiple goals, including resilience to climate change, increased carbon sequestration, and enhanced water quality (VAAFM, 2020). A legislature-mandated working group currently is working to make recommendations for a PES program.
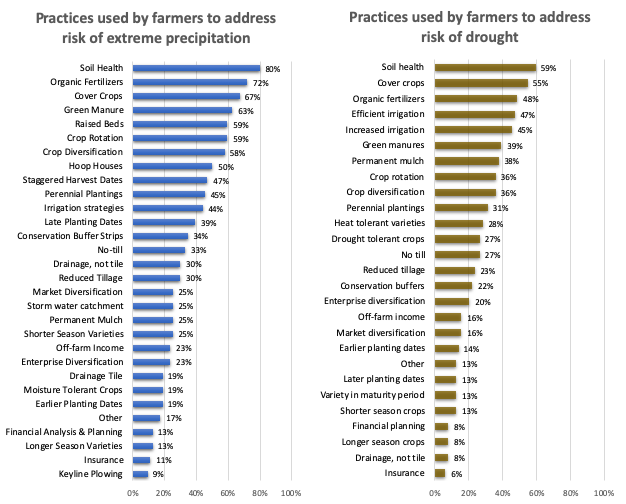
Note: Based on responses of sixty-four farmers to the New England Adaptation Survey (White et al., 2018)
5.4.4 Mitigation
Vermont farmers are aware of their environmental footprint and strive to be environmental stewards when they have the financial capacity to do so (White et al., 2018). Climate change mitigation is often considered in decisions around tractor fuel consumption, greenhouse heat sources, and purchases of agricultural plastics such as plastic mulch, irrigation equipment, and tarps. Vermont farmers have expressed a high level of interest in new programs that would compensate them to manage their farms for enhanced climate regulation or ecosystem services (White and Faulkner, 2019).
5.4.5 Seed Systems
Seed systems (formal or informal activities related to production, dissemination, and access to seeds) present a critical entry point from which broad-ranging food system challenges can be addressed. In middle- and low-income countries, informal seed systems maintained by farmers often house higher degrees of crop intra- and inter-specific diversity (Croft et al., 2018) The diversity of seeds supplies essential ecosystem services and can act as a buffer in the face of climate risks. Such ecosystem services include disease and pest suppression, resilience to climate variability, and agrobiodiversity conservation (Pautasso et al., 2013). Moreover, through continued on-farm cultivation and subsequent saving and sharing of important traditional varieties and landraces, informal seed systems allow for the continued evolution and adaptation of crops to local conditions and to changing climatic pressures (Bellon et al., 2018).
In contrast, formal seed systems are dominated by commercial seed companies and are often marked by high degrees of vertical integration, regulation, and uniformity (Gill et al., 2013). Particularly prominent in high-income countries such as the U.S., formal seed systems often perpetuate conventional agricultural practices whereby producers try to alter agricultural landscapes to model the lab conditions (fertilizers, pesticides, irrigation) in which the seeds were bred. This inadvertently leads to environmental issues (Evenson, 2003) and hinders the ability of crops to interact with symbiotic organisms (Kiers et al., 2007; Pérez-Jaramillo et al., 2019; Porter and Sachs, 2020). Seeds that flow through these systems also are often bred for specific traits, such as high yield (i.e., high yield varieties, HYV), and other traits that focus on short-term profit and lead to reduced diversity (Bellon et al., 2017). Furthermore, seeds grown in lab conditions can contribute to biotic and abiotic stress in the field because of the spatial disconnect between where they are bred (centralized location) and where they are grown (wide distribution). Continued use of formal seed systems in a changing climate will thus require more intense breeding cycles (Atlin et al., 2017). However, other models of formal seed systems do exist, in which seed companies such as High Mowing Organic Seeds in Vermont represent an alternative to conventional formal seed systems and specialize in organic and open-pollinated varieties (Helicke, 2015).
While it is true that formal systems predominate in the U.S., informal systems continue to persist in various pockets across the country (Campbell, 2012; Soleri, 2018; Veteto, 2014). Vermont is no exception, as evidenced by the prevalence of seed libraries and seed-saving groups. The Vermont Seed Systems survey showed “robust” informal seed systems in Vermont, such as the Upper Valley Seed Savers (Figure 5-9) (Baxley et al., 2020). Vermont is also home to the High Mowing Organic Seeds company, which is a prime example of a resilient and non-GMO formal seed system relative to industry giants like Monsanto. Furthermore, recent evidence indicates that non-commercial seed growers such as gardeners and seed savers may be maintaining a high degree of crop diversity (Baxley et al., 2020). As such, although public and private research communities often have centered their attention around formal systems (Otieno et al., 2017; Scoones and Thompson, 2011), informal seed systems in Vermont provide a promising agricultural area that, if supported, can provide valuable ecosystem services, bolster food security (Fess et al., 2011; Khoury et al., 2014), and assist in adapting to climate change (Ismail et al., 2013). Also, UVM is planning on saving seeds for backup and recognizes that continued cultivation and use of informal seed systems is an important way to adapt to climate change.
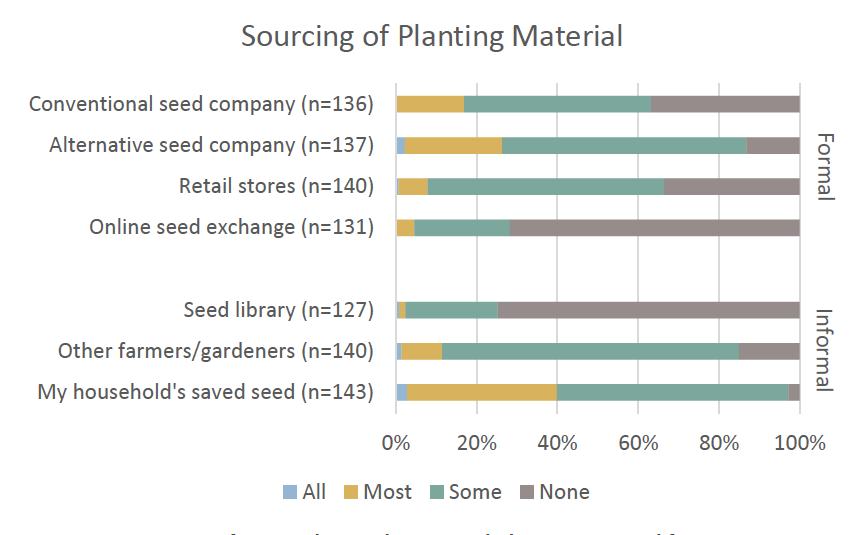
Box 5.2: Informal seed systems: A Vermont Case Study (J. Guo, 2020)
The many informal seed networks that exist in Vermont can act as a framework for sustainable, community-based food systems. One example can be found in the Bhutanese-Nepali refugee population that has settled in Chittenden County. In a study of two community garden programs, New Farms for New Americans (NFNA) at the Ethan Allen homestead (n=15) and Winooski Community Gardens (n=15), participants were found to practice sustainable, community-based farming that provided a source of culturally important foods. Although these farmers use both formal and informal seed systems, informal seed systems are an important source for culturally important varieties that are difficult to find within the formal system. Moreover, informal seed systems help maintain social relationships between family members and friends. Indeed, many NFNA farmers share seeds among each other and can take advantage of greenhouses and their own homes to extend the growing season and grow crops that are not well adapted to Vermont’s relatively short growing season. Thus, Bhutanese-Nepali refugees are not only maintaining a valuable cultural and genetic diversity through their production and subsequent seed saving but they are also adapting crops to Vermont and diversifying the gardening landscape of Chittenden County.There are many examples of how the Bhutanese-Nepali farmers have adapted to relatively Vermont’s short growing season. In their native countries, the growing season allows for some varieties of long-growing pumpkins to grow to maturity; while in Vermont they eat the leaves and shoots from such pumpkins throughout the growing season though the gourd may not reach maturity by the time the growing season ends. The community has also experimented with a traditional crop called tukruke. One farmer tried it out in Vermont, made the necessary adjustments, and then shared his seeds and knowledge with others; now it is common in the Bhutanese-Nepali plots at both the Ethan Allen and Winooski gardens.
5.5 Traceable Accounts
Traceable accounts describe the confidence level—the degree of certainty in the scientific evidence—for each key message resulting from this chapter. This analysis is based on the U.S. Global Change Research Program guidance in the Fourth National Climate Assessment (USGCRP, 2018).
Confidence level
- Very High – Strong evidence (established theory, multiple sources, confident results, well-documented and accepted methods, etc.), high consensus
- High – Moderate evidence (several courses, some consistency, methods vary, and/or documentation limited, etc.), medium consensus
- Medium – Suggestive evidence (a few sources, limited consistency, models incomplete, methods emerging, etc.), competing schools of thought
- Low – Inconclusive evidence (limited sources, extrapolations, inconsistent findings, poor documentation and/or methods not tested, etc.), disagreement or lack of opinions among experts
Key Message 1
Vermont’s climate is already changing in ways that benefit its agricultural system, including longer growing periods (freeze-free periods lengthened twenty-one days since early 1900s) and milder temperatures (increased annual average temperatures 2°F (1.1°C) since the 1990s), allowing farmers to experiment with new crops or practices not previously viable in Vermont.
- Confidence level: High
- Major uncertainties: Degree of year-to-year weather fluctuations from the normal is a global phenomenon and will be a function of GHG mitigation and sequestration.
- References: Trends in long-term Vermont-specific data. Climate Change in Vermont chapter and Wolfe et al. (2018)
Key Message 2
The changing climate causes agricultural setbacks, such as negative impacts on fruit-bearing species like apple trees that require a sufficient over-wintering period for success in the next growing season. The maple syrup industry is also at risk due to variations in winter temperatures.
- Confidence level: High
- Major uncertainties: Degree of year-to-year weather fluctuations from the normal is a global phenomenon and will be a function of GHG mitigation and sequestration.
- References: Trends in long-term Vermont-specific data. Climate Change in Vermont chapter and Wolfe et al. (2018)
Key Message 3
Climate models predict tougher growing conditions due to greater variability in temperature and precipitation, including heavy precipitation and dry spells.
- Confidence level: High
- Major uncertainties: Degree of year-to-year weather fluctuations from the normal is a global phenomenon and will be a function of GHG mitigation and sequestration.
- References: Trends in long-term Vermont-specific data. Climate Change in Vermont chapter and Wolfe et al. (2018)
Key Message 4
Vermont’s average annual precipitation has increased 6.7 inches since the 1960s. Summer precipitation has increased most (additional 2.6 inches since 1960s) and is characterized by more heavy precipitation events (defined as more than one inch of precipitation in one day) (additional 1.0 day/year), although spring precipitation has also increased notably (additional 2.11 inches/year since 1960s, and 0.8 days/year with heavy precipitation). Spring precipitation accumulates in the soil and can make farm operations difficult. While precipitation during the growing season is trending upward, precipitation falls in fewer, more extreme events and is coupled with longer periods of no rain at times when crop water requirements are still high; thus, irrigation may become increasingly important.
- Confidence level: Medium
- Major uncertainties: Degree of year-to-year weather fluctuations from the normal is a global phenomenon and will be a function of GHG mitigation and sequestration.
- References: Trends in worldwide atmospheric data. Wolfe et al. (2018)
Key Message 5
At the Earth’s surface, increasing concentrations of carbon dioxide may benefit yields in crops that utilize the C3 photosynthetic pathway (i.e., many of Vermont’s forages) if conditions are otherwise ideal. Conversely, an increase in surface-level ozone concentrations may reduce crop productivity.
- Confidence level: Medium
- Major uncertainties: Degree of year-to-year weather fluctuations from the normal is a global phenomenon and will be a function of GHG mitigation and sequestration.
- References: Trends in worldwide and Vermont-specific data and climate models. Wolfe et al. (2018)
Key Message 6
Extreme events are expected to increase, which will result in more periods of flooding and drought and crop damages or failures. Stormwater and irrigation infrastructure will be crucial in mitigating these effects.
- Confidence level: Medium
- Major uncertainties: Degree of year-to-year weather fluctuations from the normal is a global phenomenon and will be a function of GHG mitigation and sequestration.
- References: Trends in worldwide and Vermont-specific data and climate models. Wolfe et al. (2018)
Key Message 7
Agriculture and food systems may play an important role in mitigating climate change, if mitigation provides financial opportunities, are distributed fairly, and are implemented accurately with careful monitoring, reporting and verification. Urban and suburban areas in Vermont have the potential to improve adaptation and mitigation of climate change by growing food closer to where it is consumed.
- Confidence level: Medium
- Major uncertainties: Degree of year-to-year weather fluctuations from the normal is a global phenomenon and will be a function of GHG mitigation and sequestration. Public awareness and action are a function of perceived urgency to address climate related issues.
- References: Trends in worldwide and Vermont-specific data and climate models. Vermont farm research and surveys. Birthisel et al. (2020); Willard et al. (2020); Wolfe et al. (2018)
5.6 References
Armstrong, D. S., Richards, T. A., and Parker, G. W. (2001). Assessment of Habitat, Fish Communities, and Streamflow Requirements for Habitat Protection, Ipswich River, Massachusetts (Water-Resources Investigations Report No. 2001–4161). https://doi.org/10.3133/wri20014161
Atlin, G. N., Cairns, J. E., and Das, B. (2017). Rapid breeding and varietal replacement are critical to adaptation of cropping systems in the developing world to climate change. Global Food Security, 12, 31–37. https://doi.org/10.1016/j.gfs.2017.01.008
Baxley, S., Isbell, C. V., and Tobin, D. (2020). Vermont Seed Saver and Producer Survey: 2020 Summary Report. 17.
Bellon, M. R., Dulloo, E., Sardos, J., Thormann, I., and Burdon, J. J. (2017). In situ conservation-harnessing natural and human-derived evolutionary forces to ensure future crop adaptation. Evolutionary Applications, 10(10), 965–977. https://doi.org/10.1111/eva.12521
Bellon, M. R., Mastretta-Yanes, A., Ponce-Mendoza, A., Ortiz-Santamaría, D., Oliveros-Galindo, O., Perales, H., Acevedo, F., and Sarukhán, J. (2018). Evolutionary and food supply implications of ongoing maize domestication by Mexican campesinos. Proceedings of the Royal Society B: Biological Sciences, 285(1885), 20181049. https://doi.org/10.1098/rspb.2018.1049
Birthisel, S. K., Eastman, B. A., Soucy, A. R., Paul, M., Clements, R. S., White, A., Acquafredda, M. P., Errickson, W., Zhu, L.-H., Allen, M. C., Mills, S. A., Dimmig, G., and Dittmer, K. M. (2020). Convergence, continuity, and community: A framework for enabling emerging leaders to build climate solutions in agriculture, forestry, and aquaculture. Climatic Change, 162(4), 2181–2195. https://doi.org/10.1007/s10584-020-02844-w
Bradshaw, T. (2016, June 8). Fire blight symptoms appearing in area orchards. UVM Fruit Blog. https://blog.uvm.edu/fruit/2016/06/08/fire-blight-symptoms-appearing-in-area-orchards/
Campbell, B. (2012). Open-Pollinated Seed Exchange: Renewed Ozark Tradition as Agricultural Biodiversity Conservation. Journal of Sustainable Agriculture, 36(5), 500–522. https://doi.org/10.1080/10440046.2011.630776
Chakraborty, S., and Newton, A. C. (2011). Climate change, plant diseases and food security: An overview: Climate change and food security. Plant Pathology, 60(1), 2–14. https://doi.org/10.1111/j.1365-3059.2010.02411.x
City and County of Honolulu. (2019). Ola: O’ahu Resilience Strategy. https://resilientcitiesnetwork.org/downloadable_resources/Network/Honolulu-Resilience-Strategy-English.pdf
City of Oakland. (2016). Resilient Oakland: It takes a town to thrive. https://resilientcitiesnetwork.org/downloadable_resources/Network/Oakland-Resilience-Strategy-English.pdf
Croft, M. M., Marshall, M. I., Odendo, M., Ndinya, C., Ondego, N. N., Obura, P., and Hallett, S. G. (2018). Formal and Informal Seed Systems in Kenya: Supporting Indigenous Vegetable Seed Quality. The Journal of Development Studies, 54(4), 758–775. https://doi.org/10.1080/00220388.2017.1308487
Dáder, B., Fereres, A., Moreno, A., and Trębicki, P. (2016). Elevated CO2 impacts bell pepper growth with consequences to Myzus persicae life history, feeding behaviour and virus transmission ability. Scientific Reports, 6(1), 19120. https://doi.org/10.1038/srep19120
Debarro, P., and Maelzer, D. (1993). Influence of High-Temperatures on the Survival of Rhopalosiphum-Padi (L) (Hemiptera, Aphididae) in Irrigated Perennial Grass Pastures in South-Australia. Australian Journal of Zoology, 41(2), 123. https://doi.org/10.1071/ZO9930123
Eastburn, D. M., Degennaro, M. M., Delucia, E. H., Dermody, O., and Mcelrone, A. J. (2010). Elevated atmospheric carbon dioxide and ozone alter soybean diseases at SoyFACE: Atmospheric change alters diseases at SoyFACE. Global Change Biology, 16(1), 320–330. https://doi.org/10.1111/j.1365-2486.2009.01978.x
Evenson, R. E. (2003). Assessing the Impact of the Green Revolution, 1960 to 2000. Science, 300(5620), 758–762. https://doi.org/10.1126/science.1078710
Fess, T. L., Kotcon, J. B., and Benedito, V. A. (2011). Crop Breeding for Low Input Agriculture: A Sustainable Response to Feed a Growing World Population. Sustainability, 3(10), 1742–1772. https://doi.org/10.3390/su3101742
Gill, T., Bates, R., Bicksler, A., Burnette, R., Ricciardi, V., and Yoder, L. (2013). Strengthening Informal Seed Systems to Enhance Food Security in Southeast Asia. Journal of Agriculture, Food Systems, and Community Development, 139–153. https://doi.org/10.5304/jafscd.2013.033.005
Guo, H., Sun, Y., Li, Y., Liu, X., Zhang, W., and Ge, F. (2014). Elevated CO 2 decreases the response of the ethylene signaling pathway in Medicago truncatula and increases the abundance of the pea aphid. New Phytologist, 201(1), 279–291. https://doi.org/10.1111/nph.12484
Guo, J. (2020). Seed Systems of Bhutanese-Nepali Refugee Gardeners: Making Place in Chittenden County, Vermont [MS]. UVM.
Helicke, N. A. (2015). Seed exchange networks and food system resilience in the United States. Journal of Environmental Studies and Sciences, 5(4), 636–649. https://doi.org/10.1007/s13412-015-0346-5
Hirschi, M., Stoeckli, S., Dubrovsky, M., Spirig, C., Calanca, P., Rotach, M. W., Fischer, A. M., Duffy, B., and Samietz, J. (2012). Downscaling climate change scenarios for apple pest and disease modeling in Switzerland. Earth System Dynamics, 3(1), 33–47. https://doi.org/10.5194/esd-3-33-2012
Ismail, A. M., Singh, U. S., Singh, S., Dar, M. H., and Mackill, D. J. (2013). The contribution of submergence-tolerant (Sub1) rice varieties to food security in flood-prone rainfed lowland areas in Asia. Field Crops Research, 152, 83–93. https://doi.org/10.1016/j.fcr.2013.01.007
Kavet. (2020). The Vermont Farming, Food Production, and Food Distribution Industries, 2020 Update.
Khoury, C. K., Bjorkman, A. D., Dempewolf, H., Ramirez-Villegas, J., Guarino, L., Jarvis, A., Rieseberg, L. H., and Struik, P. C. (2014). Increasing homogeneity in global food supplies and the implications for food security. Proceedings of the National Academy of Sciences, 111(11), 4001–4006. https://doi.org/10.1073/pnas.1313490111
Kiers, E. T., Hutton, M. G., and Denison, R. F. (2007). Human selection and the relaxation of legume defences against ineffective rhizobia. Proceedings of the Royal Society B: Biological Sciences, 274(1629), 3119–3126. https://doi.org/10.1098/rspb.2007.1187
King, K. W., Williams, M. R., Macrae, M. L., Fausey, N. R., Frankenberger, J., Smith, D. R., Kleinman, P. J. A., and Brown, L. C. (2015). Phosphorus Transport in Agricultural Subsurface Drainage: A Review. Journal of Environmental Quality, 44(2), 467–485. https://doi.org/10.2134/jeq2014.04.0163
Lin, X., Ruess, P. J., Marston, L., and Konar, M. (2019). Food flows between counties in the United States. Environmental Research Letters, 14(8), 084011. https://doi.org/10.1088/1748-9326/ab29ae
Madramootoo, C. A. (1999). Planning and Design of Drainage Systems. In R. W. Skaggs and J. van Schilfgaarde (Eds.), Agronomy Monographs (pp. 871–892). American Society of Agronomy, Crop Science Society of America, Soil Science Society of America. https://doi.org/10.2134/agronmonogr38.c26
Mase, A. S., Gramig, B. M., and Prokopy, L. S. (2017). Climate change beliefs, risk perceptions, and adaptation behavior among Midwestern U.S. crop farmers. Climate Risk Management, 15, 8–17. https://doi.org/10.1016/j.crm.2016.11.004
McCallum, K. (2020). Drought Disaster Declared for 10 Vermont Counties | Off Message [News]. Seven Days VT. https://www.sevendaysvt.com/OffMessage/archives/2020/11/17/drought-disaster-declared-for-10-vermont-counties
Moore, J. (2016). Literature Review: Tile Drainage and Phosphorus Losses from Agricultural Land (Technical Report No. 83; p. 79). Stone Environmental.
Moretti, C. L., Mattos, L. M., Calbo, A. G., and Sargent, S. A. (2010). Climate changes and potential impacts on postharvest quality of fruit and vegetable crops: A review. Food Research International, 43(7), 1824–1832. https://doi.org/10.1016/j.foodres.2009.10.013
National Integrated Drought Information System. (n.d.). Drought in Vermont from 2000-Present. National Integrated Drought Information System. Retrieved March 12, 2021, from https://www.drought.gov/states/vermont#:~:text=Drought%20in%20Vermontandtext=The%20U.S.%20Drought%20Monitor%20started,affected%2029.39%25%20of%20Vermont%20land
Nickerson, G., Grunbinger, V., Nwadike, L., and Blevins, L. (2013). Frequently asked questions about handling flooded produce (p. 8). University of Vermont.
Oehme, V., Högy, P., Zebitz, C. P. W., and Fangmeier, A. (2013). Effects of elevated atmospheric CO 2 concentrations on phloem sap composition of spring crops and aphid performance. Journal of Plant Interactions, 8(1), 74–84. https://doi.org/10.1080/17429145.2012.736200
Otieno, G. A., Reynolds, T. W., Karasapan, A., and Noriega, I. L. (2017). Implications of Seed Policies for On-Farm Agro-Biodiversity in Ethiopia and Uganda. Sustainable Agriculture Research, 6(4), 12. https://doi.org/10.5539/sar.v6n4p12
Pautasso, M., Aistara, G., Barnaud, A., Caillon, S., Clouvel, P., Coomes, O. T., Delêtre, M., Demeulenaere, E., De Santis, P., Döring, T., Eloy, L., Emperaire, L., Garine, E., Goldringer, I., Jarvis, D., Joly, H. I., Leclerc, C., Louafi, S., Martin, P., … Tramontini, S. (2013). Seed exchange networks for agrobiodiversity conservation. A review. Agronomy for Sustainable Development, 33(1), 151–175. https://doi.org/10.1007/s13593-012-0089-6
Pérez-Jaramillo, J. E., de Hollander, M., Ramírez, C. A., Mendes, R., Raaijmakers, J. M., and Carrión, V. J. (2019). Deciphering rhizosphere microbiome assembly of wild and modern common bean (Phaseolus vulgaris) in native and agricultural soils from Colombia. Microbiome, 7(1), 114. https://doi.org/10.1186/s40168-019-0727-1
Porter, S. S., and Sachs, J. L. (2020). Agriculture and the Disruption of Plant–Microbial Symbiosis. Trends in Ecology and Evolution, 35(5), 426–439. https://doi.org/10.1016/j.tree.2020.01.006
Raza, A., Razzaq, A., Mehmood, S., Zou, X., Zhang, X., Lv, Y., and Xu, J. (2019). Impact of Climate Change on Crops Adaptation and Strategies to Tackle Its Outcome: A Review. Plants, 8(2), 34. https://doi.org/10.3390/plants8020034†
Robinson, E. A., Ryan, G. D., and Newman, J. A. (2012). A meta‐analytical review of the effects of elevated CO2 on plant–arthropod interactions highlights the importance of interacting environmental and biological variables. New Phytologist, 194(2), 321–336. https://doi.org/10.1111/j.1469-8137.2012.04074.x
Scoones, I., and Thompson, J. (2011). The Politics of Seed in Africa’s Green Revolution: Alternative Narratives and Competing Pathways. IDS Bulletin, 42(4), 1–23. https://doi.org/10.1111/j.1759-5436.2011.00232.x
Soleri, D. (2018). Civic seeds: new institutions for seed systems and communities—a 2016 survey of California seed libraries. Agriculture and Human Values, 35(2), 331–347. https://doi.org/10.1007/s10460-017-9826-4
Stafne, E. T. (2020). Chilling-Hour Requirements of Fruit Crops. 2.
Tan, C. S., and Zhang, T. Q. (2011). Surface runoff and sub-surface drainage phosphorus losses under regular free drainage and controlled drainage with sub-irrigation systems in southern Ontario. Canadian Journal of Soil Science, 91(3), 349–359. https://doi.org/10.4141/cjss09086
Trębicki, P., and Finlay, K. (2018). Pests and Diseases under Climate Change; Its Threat to Food Security. In S. S. Yadav, R. J. Redden, J. L. Hatfield, A. W. Ebert, and D. Hunter, Food Security and Climate Change (pp. 229–249). John Wiley and Sons, Ltd. https://doi.org/10.1002/9781119180661.ch11
Trenberth, K. (2011). Changes in precipitation with climate change. Climate Research, 47(1), 123–138. https://doi.org/10.3354/cr00953
USGCRP (2018). Impacts, Risks, and Adaptation in the United States: Fourth National Climate Assessment, Volume II: [Reidmiller, D.R., C.W. Avery, D.R. Easterling, K.E. Kunkel, K.L.M. Lewis, T.K. Maycock, and B.C. Stewart (eds.)]. U.S. Global Change Research Program, Washington, DC, USA, 1515 pp. doi: 10.7930/NCA4.2018.
USGS. (2021a). Downscaled Climate Change Modeling for the Conterminous United States (National Assessment). https://www.usgs.gov/centers/casc-sc/science/downscaled-climate-change-modeling-conterminous-united-states-national?qt-science_center_objects=0#qt-science_center_objects
USGS. (2021b). USGS Current Water Data for Vermont. https://waterdata.usgs.gov/vt/nwis/rt
VAAFM (Vermont Agency of Agriculture, Food and Markets). (2020). Soil conservation practices and payment for ecosystem services working group report. Prepared for the Vermont General Assembly in Accordance with Act No. 83, Section 3 (p. 44). Vermont Agency of Agriculture, Food and Markets.
Veteto, J. R. (2014). Seeds of Persistence: Agrobiodiversity in the American Mountain South. Culture, Agriculture, Food and Environment, 36(1), 17–27. https://doi.org/10.1111/cuag.12026
White, A. (2021, December 19). The Use of Crop Insurance for Climate Resilience on Vegetable and Fruit Farms in the Northeast: Insights from the New England Adaptation Survey. https://na.eventscloud.com/file_uploads/a1665c86ae3c21daf15709fc882284ef_White_NEAdaptationSurveyWaterSymposium1.pdf
White, A., and Faulkner, J. (2019). Enhancing participation in payment for ecosystem services programs: Understanding farmer perspectives. https://www.uvm.edu/sites/default/files/The-Center-for-Sustainable-Agriculture/farmer_perspectives_on_PES_AW_JF_working_version.pdf
White, A., Faulkner, J., Sims, S., Tucker, P., and Weatherhogg, K. (2018). The 2017-2018 New England Adaptation Survey for Vegetable and Fruit Growers: Preliminary Results (p. 38) [Survey]. University of Vermont.
White, A., and Pankoff, A. (2019). Resources for climate resilience: Listening tour with 173 farmers from Pennsylvania to Prince Edward Island [Unpublished Raw Data].
Willard, A., Harris, K., Kahler, E., Claro, J., Danly, S., and Warren, B. (2020). Vermont Agriculture and Food System Plan: 2020. 110. https://agriculture.vermont.gov/document/vermont-agriculture-and-food-system-plan-2020
Wolfe, D. W., DeGaetano, A. T., Peck, G. M., Carey, M., Ziska, L. H., Lea-Cox, J., Kemanian, A. R., Hoffmann, M. P., and Hollinger, D. Y. (2018). Unique challenges and opportunities for northeastern US crop production in a changing climate. Climatic Change, 146(1–2), 231–245. https://doi.org/10.1007/s10584-017-2109-7